Evolution of nanostructured materials produced by separate electrochemical oxidation of copper and aluminum
Автор: Usoltseva N.V., An V.V., Damdinov B.B.
Журнал: Журнал Сибирского федерального университета. Серия: Техника и технологии @technologies-sfu
Рубрика: Исследования. Проектирование. Опыт эксплуатации
Статья в выпуске: 5 т.16, 2023 года.
Бесплатный доступ
This paper covers the results of the composition and structure evolution of nanostructured materials produced by separate electrochemical oxidation of metals (copper and aluminum). The electrolysis products after short-term and long-term aging were characterized by XRD (X-ray diffraction) and DSC (differential scanning calorimetry) analysis. There is the difference in aging of nanostructures of copper- and aluminum-compounds. Short-term aging results in the phase transformation of copper(I) oxide and the stability of aluminum oxyhydroxide (boehmite). Copper (I) oxide is oxidized to copper (II) oxide and copper carbonate hydroxide. At long-term aging the oxidation of copper (I) oxide does not completed because the Pilling-Bedworth ratio for copper (II) oxide, copper carbonate hydroxide is greater than one. The structure of all copper-containing compounds (copper (I) oxide, copper (II) oxide, copper carbonate hydroxide) is changed. It results in the increases of both the interplanar spacing and the temperature of the phase transformations. Coherent scattering region (CSR) of boehmite and copper (I) oxide are 3-4 nm and 20-30 nm, respectively, and does not change at short-term and long-term aging.
Electrolysis, nanomaterials, phase composition, aging
Короткий адрес: https://sciup.org/146282708
IDR: 146282708
Текст научной статьи Evolution of nanostructured materials produced by separate electrochemical oxidation of copper and aluminum
Благодарности. Исследование проводилось с использованием оборудования Центра совместного использования «Наноматериалы и нанотехнологии» Томского политехнического университета при поддержке проекта Министерства образования и науки РФ № 075–15–2021–710.
Цитирование: Усольцева, Н. В. Эволюция наноструктурированных продуктов индивидуального электрохимического окисления меди и алюминия / Н. В. Усольцева, В. В. Ан, Б. Б. Дамдинов. Журн. Сиб. федер. ун-та. Техника и технологии, 2023, 16(5). С. 550–558. EDN: SQBFQO
The use of alternating current to carry out the electrochemical metals oxidation results in nanostructured oxides and multicomponent nanostructured oxide materials [2].
There are a number of electrochemical methods to produce coatings of metal oxides [3], mixed oxides [4], layered double hydroxides [5] and perovskites [6].
It is known that nanomaterials are characterized by interaction with compounds contained in mother solution or in the environment. New phases are formed; they may be of interest not only for science, but also for practical application [7–9].
There are the different methods of analysis to characterize the nanostructures of oxides. The choice of method depends on what information is to be obtained. To evaluate the phase compositions and coherent scattering regions (CSR), X-ray diffraction (XRD) is used. The temperatures of phase and structure transformations are provided by analysis of differential scanning calorimetry and thermogravimetric analysis (DSC/TG) [10]. The specific properties of matter at the nanoscale level are the reason of the difference the methods that can be applied to carry out the thermal analysis both bulk and dispersed materials [11].
The purpose of this work is to study the influence aging on composition and properties of nanostructured powders produced by separate electrochemical oxidation of copper and aluminum.
Research methodologies
Electrochemical oxidation of metals was carried out in a 3 wt% sodium chloride solution at the densities of alternating current of 1 A/cm 2 . Metal plates (copper or aluminum) were used as soluble electrodes.
X-ray diffraction (XRD) measurements were performed at 30 mA and 40 kV (a Shimadzu XRD 7000 diffractometer, CuK α radiation, λ=1.5418 Å). The range of 2θ is from 10 to 70°, counting rate is 2θ min -1 . The phase compositions were identified by PDF 2 database, the coherent scattering regions (CSR) were determined with the program “Powder Cell 2.4”.
SDT Q600 V20.9 Build 20 was used to carry out thermogravimetric analysis (TGA) and differential scanning calorimetry (DSC) in air at a heating rate of 10 °C min -1 in the temperature range from ambient to 1000 °C.
Results and discussion
Electrochemical copper oxidation
The rate of electrochemical copper oxidation is 0.04 g/(cm 2 ·h). Phase composition of nanostructured powder of copper (I) oxide was detected by XRD analysis. At the aging the copper (I) oxide is oxidized to copper (II) oxide, and the copper carbonate hydroxide (Cu 2 (OH) 2 CO 3 ) is formed [12].
Short-term aging (30 and 45 days) leads to an increase in the background line of XRD patterns due to amorphization of the structure during the formation of new phases. The result of the long-term aging (5 years) is the change of the structure (crystallization of the amorphous phase), because of this the background of XRD patterns becomes smoother and intensity of some Cu 2 (OH) 2 CO 3 peaks are increased (Fig. 1).
After aging for 60 days (Fig. 1, short-term aging), there is a small amount of Cu 2 O in sample. According to the XRD results, the residual Cu 2 O is not completely oxidized to CuO at long-term aging. This may be due to the fact that Cu 2 O is located in the centers of particles coated with CuO that prevents oxygen access to Cu 2 O.
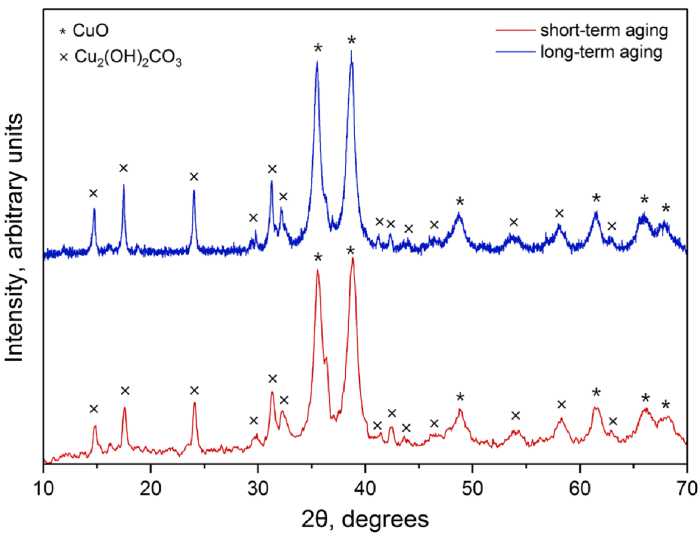
Fig. 1. XRD patterns of the nanostructured powders produced by electrochemical oxidation of copper
The protective effect of oxidation products depends on their structure, which is determined by the ratio of the volumes of oxidized and formed compounds (Pilling–Bedwords ratio / P–B ratio) [13], as well as the structure of oxidation products.
In this paper the Pilling – Bedwords ratio is determined by the following formulas:
MCuO У
_ VquO _ /Рс»О _ ^CuO ‘ A?u2O
^СиО//
/Си2° VCU2O n.MCu2<V n• MCU2O • /?СиО / Pc^O
Vcu,(OH)2CO3
Мси2(ОН)2СО3 ' А и2О
П ' МСи2о ■ Рси2 (ОН)2 со3
Мси2(ОН)2СО3 " РсиО
П ' МСио ■ Рси2 (ОН)2 со3
^Си2 (ОН)2 СОз/ -~
/Си2О*Си
Vcu2(OH)2CO3
^Си2 (ОН)2 со3 / -—
/СиО VCu0
where МсиО, Мси2О, МСи2(ОН)2СО – molar mass of CuO, Cu 2 O and Cu 2 (OH) 2 CO 3 respectively, g/mol; РсиО, Рси2О, Рси2(ОН)2СО3 – density of CuO, Cu 2 O and Cu 2 (OH) 2 CO 3 respectively, g/сm 3 ; (Рси2О = 6.11 g/сm 3 , РсиО = 6.45 g/сm 3 , Рси2(ОН)2СО3 = 4.00 g/сm 3 [14]); n – number of atoms of the copper compound per 1 molecule of the aging product.
If the Pilling–Bedworth ratio is in the range from 1 to 2, the coating is passivating and provides a protecting effect against further surface oxidation [13]. The Pilling–Bedworth ratio is greater than one for copper compounds (аСиО/Си2О — 1.05, аСи2(ОН)2СО3/Си2О - 2.35, аСи2(ОН)2СОз/СиО — 2.24). Hence, the volume of CuO or Cu 2 (OH) 2 CO 3 is larger than the volume of Cu 2 O, and the oxidation products form a continuous layer on the surface of the initial oxide. At the same time, due to the small particle size, high porosity, and nonstoichiometry of the oxidation products, the oxidation process does not stop after the formation of a thin layer of oxidation products. A small amount of Cu 2 O after longterm aging probably remains in the centers of the large particles covered by thick layer.
The Scherrer equation [1] was used to calculate the coherent scattering region (CSR):
kA d-----, P cos 0
where k is the Scherrer constant; λ is the wave length of the X-ray beam used (1.5418 Å); β is the full width at half maximum (FWHM) of the peak; Θ is the Bragg angle.
At both short-term and long-term aging CSR of boehmite and copper (I) oxide are 3–4 nm and 20–30 nm, respectively [2].
There is a XRD peak shift of all copper-containing compounds (copper (I) oxide, copper (II) oxide and copper carbonate hydroxide Cu 2 (OH) 2 CO 3 ) to the large values of the interplanar distance (Tables 1–3). The increase in interplanar distances is a result of the interaction of nanostructures with oxygen and CO 2 of the atmosphere.
The aging results in a temperature increase of the phase transformations (Fig. 2).
The increase of endothermic effects (peak I ) of dehydration as the ageing result is due to structure ordering.
The small amount of Cu 2 O cannot be identified by XRD analysis but it is detected by DSC measurements. At the temperature increase, Cu 2 O is oxidized to CuO and Cu 2 (OH) 2 CO 3 is decomposed. In the temperature range of 150–280 °C, there is an overlap of the exoeffect from the oxidation of Cu 2 O and the endoeffect from the first stage of decomposition of Cu 2 (OH) 2 CO 3 (peak II ). When Cu 2 O is oxidized at long-term aging, the Cu 2 O content decreases and the exoeffect is change in the same way.
Table 1. Interplanar spacing of copper (I) oxide
Sample |
Interplanar spacing of copper (I) oxide, nm |
|||
(110) |
(111) |
(200) |
(220) |
|
PDF No. 05–0667 |
0.3020 |
0.2465 |
0.2135 |
0.1510 |
5 days |
0.3017 |
0.2466 |
0.2135 |
0.1508 |
30 days |
0.3017 |
0.2458 |
0.2131 |
0.1508 |
45 days |
0.3024 |
0.2462 |
0.2131 |
0.1508 |
60 days |
0.3029 |
0.2488 |
0.2134 |
0.1509 |
5 years |
0.3040 |
0.2481 |
0.2136 |
0.1510 |
Table 2. Interplanar spacing of copper (II) oxide
Sample |
Interplanar spacing of copper (II) oxide, nm |
|||
(111) |
(–111) |
(–202) |
(–222) |
|
PDF No. 05–0661 |
0.2524 |
0.2311 |
0.1867 |
0.1375 |
5 days |
0.2523 |
0.2321 |
– |
– |
30 days |
0.2523 |
0.2317 |
0.1866 |
0.1372 |
45 days |
0.2525 |
0.2317 |
0.1866 |
0.1375 |
60 days |
0.2525 |
0.2320 |
0.1868 |
0.1380 |
5 years |
0.2527 |
0.2328 |
0.1870 |
0.1393 |
Table 3. Interplanar spacing of copper carbonate hydroxide
Sample |
Interplanar spacing of copper carbonate hydroxide, nm |
|||||||
(020) |
(120) |
(220) |
(20–1) |
(21–1) |
(041) |
(250) |
(061) |
|
PDF No. 41–1390 |
0.5970 |
0.5040 |
0.3690 |
0.2861 |
0.2781 |
0.2185 |
0.2128 |
0.1689 |
5 days |
– |
– |
– |
– |
– |
– |
– |
– |
30 days |
0.5990 |
0.5048 |
0.3691 |
0.2853 |
0.2747 |
0.2183 |
0.2131 |
0.1705 |
45 days |
0.5990 |
0.5048 |
0.3691 |
0.2849 |
0.2770 |
0.2183 |
0.2131 |
0.1683 |
60 days |
0.5990 |
0.5048 |
0.3697 |
0.2858 |
0.2762 |
0.2182 |
0.2134 |
0.1691 |
5 years |
0.6018 |
0.5071 |
0.3705 |
0.2860 |
0.2785 |
0.2196 |
0.2136 |
0.1691 |
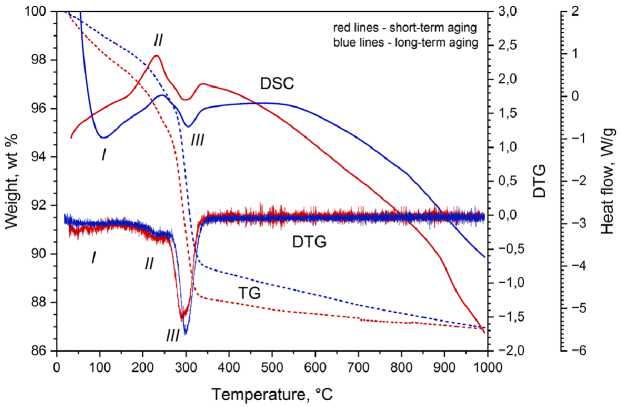
Fig. 2. DSC/DTG/TG curves of nanostructures produced by non-equilibrium electrochemical copper oxidation
The temperature of Cu 2 O oxidation to CuO (peak II ) changes from 231.3 to 245.2 ºC whereas the temperature of Cu 2 (OH) 2 CO 3 decomposition changes from 298.2 to 304.7 ºC (peak III ).
The overall weight loss of nanostructures of copper compounds is 13 wt.% after both short-term and long-term aging. Comparison of the weight loss integral and differential curves reveals that after long-term aging the temperature range of the second Cu 2 (OH) 2 CO 3 decomposition stage is narrower than at short-term aging. Because of this, it seems that long-term aging causes greater weight loss during the Cu 2 (OH) 2 CO 3 decomposition.
Electrochemical aluminum oxidation
The structure of aluminum oxidation product (boehmite AlOOH, No. 17–0940) is not significantly changed at long-term aging (Fig. 3). Also, the shape and width of the peaks is not changed. The difference in the intensities of the (120), (031), (051) peaks indicates the anisotropy of the particles. As a result, the samples used to XRD analysis consist of the particles with different dominant faces.
Comparison of TG/DTG/DSC curves during heat treatment of the products of aluminum electrochemical oxidation is shown in Figure 4. At short-term aging, weight loss is 24.87 %. At – 555 –
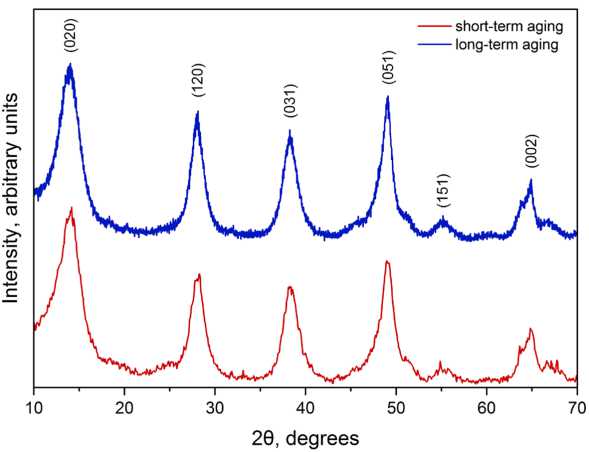
Fig. 3. XRD patterns of the nanostructured powders produced by electrochemical oxidation of aluminum
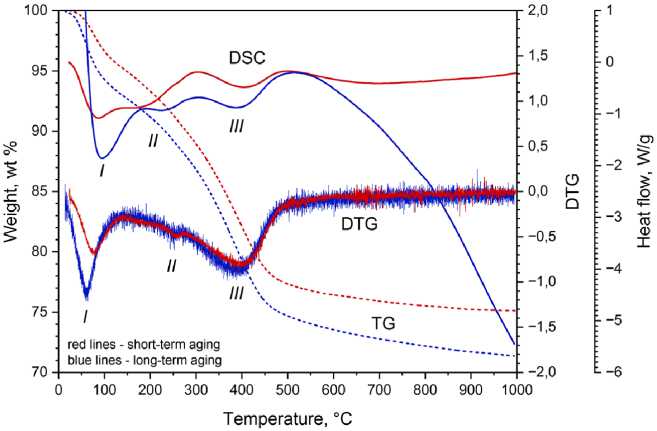
Fig. 4. DSC/DTG/TG curves of nanostructures produced by non-equilibrium electrochemical aluminum oxidation
Table 4. Endothermic effects of DSC curves of boehmite
The sharpest weight change is observed at temperatures up to 100 °C. Endothermic effects (peak I ) at a temperature of 72 °C (short-term aging) and 94 °C (long-term aging) correspond to this weight loss. The removal of moisture at higher temperatures is the result of decrease in the reactivity of the nanomaterial due to a change in the structure ordering. The comparison of the heat flow reveals that the temperatures of boehmite decomposition increase by 20–45 °C after long-term aging (Table 4, peaks II and III ). It is due to some transformations of nanostructured products of electrochemical aluminum oxidation.
Conclusions
In this paper, the evolution of the nanostructured materials produced by separate electrochemical oxidation of copper and aluminum has been considered. The XRD measurements showed a peak shift of all copper-containing compounds (copper (I) oxide, copper (II) oxide and copper carbonate hydroxide Cu 2 (OH) 2 CO 3 ) to the large values of the interplanar distance. It has been assumed that the increase in interplanar distances is related to the interaction of nanostructures with oxygen and CO 2 of the atmosphere. TG/DTG/DSC measurements of the aluminum electrochemical oxidation products showed that the weight loss is 24.87 % at short-term aging, whereas it increases to 28.63 %. at longterm aging because of physical sorption of atmospheric moisture and compounds. Transformations of nanostructured products of electrochemical aluminum oxidation can cause an increase by 20– 45 °C in the temperature of boehmite decomposition after long-term aging. Generally, we can state a significant evolution of the products of separate copper and aluminum electrochemical oxidation over time.
Список литературы Evolution of nanostructured materials produced by separate electrochemical oxidation of copper and aluminum
- Baig N., Kammakakam I., Falath W. Nanomaterials: a review of synthesis methods, properties, recent progress, and challenges, Materials Advances, 2021, 6(2), 1821-1871
- Korobochkin V. V., Potgieter J. H., Usoltseva N. V., Dolinina A. S., An V. V. Thermal preparation and characterization of nanodispersed copper-containing powders produced by non-equilibrium electrochemical oxidation of metals, Solid State Sciences, 2020, 108, article number 106434
- Zubairu A., Gimba A. S.B., Gutti B., Audu A., Potentiostatic Electro-Deposition ofp-n Homo-Junction Cuprous Oxide Solar Cells, Energy and Power, 2020, 10, 20-25
- Dresvyannikov A. F., Petrova E. V., Khairullina A. I. Electrochemical Synthesis and Physicochemical Properties of Nanostructured Al2O3-ZrO2-MgO Oxide Systems, Protection of Metals and Physical Chemistry of Surfaces, 2020, 56, 89-93
- Musella E., Gualandi I., Scavetta E., Rivalta A., Venuti E., Christian M., Morandi V., Mullaliu A., Giorgetti M., Tonelli D. Newly developed electrochemical synthesis of Co-based layered double hydroxides: toward noble metal-free electro-catalysis, J. Mater. Chem. A., 2019, 7, 11241-11249
- Atta N. F., Galal A. El-Ads E. H. Perovskite Nanomaterials - Synthesis, Characterization, and Applications, in: Perovskite Materials, IntechOpen, 2016, 107-151
- Mishra A. K., Roldan A., de Leeuw N. H. CuO Surfaces and CO2 Activation: A Dispersion-Corrected DFT+^ Study, The Journal of Physical Chemistry, C, 2016, 120(4), 2198-2214
- Wan Nor Roslam Wan Isahak, Zatil Amali CheRamli, Mohamad Wafiuddin Ismail, Khomah Ismail, Rahimi M. Yusop, Mohamed Wahab Mohamed Hisham, Mohd Ambar Yarmo, Adsorption-desorption of CO2 on different type of copper oxides surfaces: Physical and chemical attractions studies, Journal of CO2 Utilization, 2013, 2, 8-15
- Su Ch., Suarez D. L. In situ infrared speciation of adsorbed carbonate on aluminum and iron oxides, Clays and Clay Minerals, 1997, 45(6), 814-825
- Akash M. S.H., Rehman K. Introduction to Thermal Analysis, in: Essentials of Pharmaceutical Analysis, Springer, Singapore, 2020, 195-198
- Mansfield E. Recent advances in thermal analysis of nanoparticles: methods, models and kinetics, Modeling, Characterization, and Production of Nanomaterials. Edited by V. K. Tewary, Y. Zhang, Woodhead Publishing, 2015, 167-178
- Усольцева Н. В., Коробочкин В. В., Балмашнов М. А., Богодяж Ю. Е. Получение оксидов меди методом электрохимического синтеза в нейтральной среде под действием переменного тока. Промышленная химия и катализ: сборник научных трудов. Под ред. В. Н. Грунского. М. РХТУ им. Д. И. Менделеева, 2012, 185, 115-120 [Usoltseva N. V., Korobochkin V. V., Balmashnov M. A., Bogodyazh Yu. E. Production of copper oxides by electrochemical synthesis in a neutral medium under the action of alternating current. Industrial chemistry and catalysis: a collection of scientific papers. Moscow, RCTU named after D. I. Mendeleev, 2012, 185, 115-120 (in Russian)]
- McCafferty E. Introduction to Corrosion Science. Springer Science+Business Media, 2010, New York, 575 p.
- Рабинович В. А., Хавин З. Я. Краткий химический справочник. Ленинград: Химия, 1978, 392 с. [Rabinovich V. A., Khavin Z. Ya. Brief chemical reference book. Leningrad, Chemistry, 1978, 392 p. (in Russian)]