Evolution of phase composition of composite materials at contact of titanium-aluminium obtained by welding explosion
Автор: Zakharova Yelena V., Alekseeva Ksenya V., Zhmak Yelena G.
Журнал: Журнал Сибирского федерального университета. Серия: Техника и технологии @technologies-sfu
Статья в выпуске: 4 т.12, 2019 года.
Бесплатный доступ
In this work, welding explosion of multiple sample with its further annealing to 300 degrees is used as the method of receiving layered materials. Using this technology allows heating in the air; herewith possibility of oxygen penetration into internal material layers is eliminated. Using this technology allows heating in the air. Furthermore, pressures developed in the process of welding exposure ensure quality contact between the surfaces of plates [1].
Titanium-aluminium, composite materials, phase transformation, welding explosion, intermetallides
Короткий адрес: https://sciup.org/146281204
IDR: 146281204 | DOI: 10.17516/1999-494X-0145
Текст научной статьи Evolution of phase composition of composite materials at contact of titanium-aluminium obtained by welding explosion
At the moment, one of the most important tasks of machinery manufacturing development is improvement of quality, reliability and lifetime of the components of various machines and mechanisms. For solving this problem complex approach, including creation of new materials, development and learning to use new technologies is needed. The work [2] represents the method of obtaining composite materials on the basis of Ti-Al.
Many papers are concerned with structural transformation in the alloys on the basis of aluminum and titanium. Titanium alloys are characterized by sufficient specific strength, high anticorrosion properties and considerable heat resistance. The advantage of heat-resistant Titanium alloys is small specific weight and small specific stress during operation of the components in centrifugal conditions. These are disks, blades and other components of gas turbines [3]. The studies undertaken earlier showed possible simplifying the technical process of creation of layered intermetallide Ti-Al composite materials in consequence of Ti and Al melt interaction [4].
Introduction of aluminum into technical titanium even in small quantities (up to 13%) allows sharp increasing alloy heat resistance when decreasing its density and cost. This alloy is a perfect construction material. Adding 3-8% of Al increases temperature of α-Ti transformation into β-Ti. Aluminum is basically the only alloying stabilizer of α-Al increasing its strength at stability of properties of plasticity and viscosity of titanium alloy and increasing its heat resistance, creeping strength and elastic modulus. The only disadvantage of titanium is eliminated this way [5].
However, during further annealing this material might have undesirable properties, such as fragility, small strength and plasticity, in consequence of which absolutely major defect – cracks occurs [6, 7].
Purpose of work
Researching of structure and phase composition at contact of AL-Ti material, obtained by welding explosion and further annealing at 300 °C.
Samples and methods of samples obtaining
Studying material was obtained by welding explosion. 12 plates of aluminium and 11 of titanium with thickness 0.5 mm and 1 mm were used for welding explosion. Explosive material is ammonite 6LS. Welding process was made in accordance with method, which described in work [1]. Phase composition of obtained composite (point TiAl3001 in Fig. 1) was studied by X-Ray diffractometer “Bruker”. After that sample was heated in high temperature X-ray diffractometer attachment to 300 °C (point TiAl3001 in Fig. 1). After that sample was annealed for 1 hour (point TiAl3002 in Fig. 1). Results of X-ray diffraction analysis at these points are shown in Fig. 1 and Fig. 2, respectively.
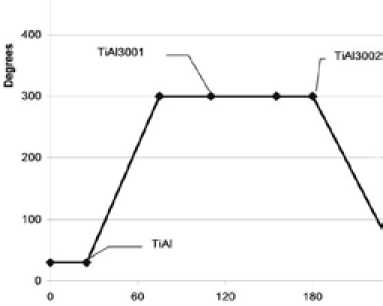
Fig. 1. Annealing scheme in X-ray diffractometer of the AL-Ti composite at 300 °C
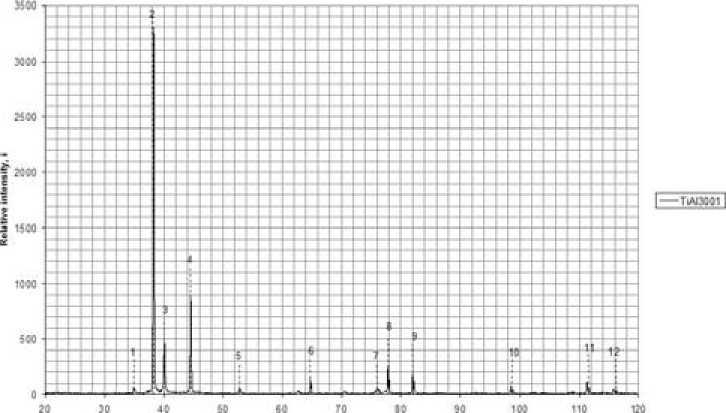
Fig 2. Diffractogram of sample of Ti-Al after heating for 1 hour to 300 °C
Results and discussion
Fig. 2 shows the X-ray diffraction pattern of Ti-Al obtained by diffraction analysis method.
As shown in Table 1, phase of solid solution of aluminum with titanium exists. According to work [8] phase reflexes of Al 3 Ti are displaced phase reflexes of Al 2 Ti are closer to the values obtained by us.
As shown in Table 2, phase TiO 2 appeared. And also a phase based on the fcc lattice of Al, which is equivalent of solid solution of aluminum with titanium, as mentioned earlier.
Welding explosion process is nonequilibrium process, as result of this process atomic displacement occurred. Such displacements were a reason of several phases’ formation i.e. nonequilibrium structure, the positions of the atoms in this structure do not exactly correspond to the interatomic distances of equilibrium structures. The estimation of such displacements is given in the modifications column in Table 1.
Table 1. Explanation of the X-ray diffraction pattern shown in Fig. 2
№ |
Ti cph |
Al face-centered |
Θ |
d (hkl) |
2 Θ |
Al 3 Ti Pm3m |
Al 2 Ti ГПУ |
Modifications |
||
hkl |
d(hkl) |
hkl |
d(hkl) |
|||||||
1 |
100 |
2.56437 |
17.6 |
2.5477 |
35.2 |
3.80 |
3.94 |
+1.3923 |
||
2 |
111 |
2.34925 |
19.15 |
2.3485 |
38.3 |
2.196 |
2.277 |
-0.0715 |
||
3 |
101 |
2.24902 |
20.05 |
2.2468 |
40.1 |
2.714 |
2.814 |
+0.5672 |
||
4 |
200 |
2.03495 |
22.25 |
2.0343 |
44.5 |
1.9 |
1.97 |
-0.0643 |
||
5 |
102 |
1.73022 |
26.45 |
1.7293 |
52.9 |
1.696 |
1.758 |
+0.0287 |
||
6 |
1.47892 |
220 |
1.43858 |
32.35 |
1.4395 |
64.7 |
1.342 |
1.392 |
-0.0475 |
|
7 |
112 |
1.25098 |
38.1 |
1.2484 |
76.2 |
1.551 |
1.608 |
+0.3596 |
||
8 |
311 |
1.22657 |
38.9 |
1.2267 |
77.8 |
1.145 |
1.187 |
-0.0397 |
||
9 |
222 |
1.17432 |
41 |
1.1741 |
82 |
1.098 |
1.138 |
-0.0361 |
||
10 |
400 |
1.01681 |
49.25 |
1.0168 |
98.5 |
0.95 |
0.985 |
-0.0318 |
||
11 |
331 |
0.93297 |
55.65 |
0.9330 |
11.3 |
0.871 |
0.903 |
-0.03 |
||
12 |
420 |
0.90946 |
58 |
0.9083 |
116 |
0.850 |
0.881 |
-0.0273 |
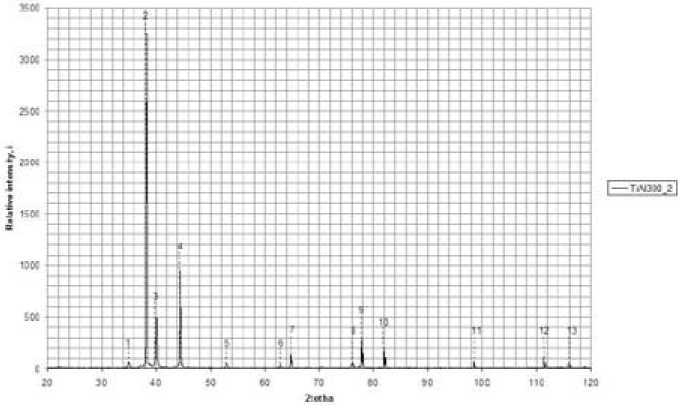
Fig. 3. Diffractogram of sample of Ti-Al after curing for 1 hour at temperature of 300 °C
As shown in Table 3, new lines № 6, № 8, № 9 appeared, explanations of these lines determined the Al 2 Ti phase with a hcp lattice at 300 °C.
The explanations showed that Al3Ti coincides within the error limits with the results of work [7, 8]. The detection of the Al 2 Ti phase coincides with the results presented in works [7] and [9].
Fig. 4 shows transformation way from the bcc lattice to the hcp lattice with insignificant atomic displacements through the fcc phase.
Conclusions
-
1) Annealing at 300 °С temperature were a reason of titanium oxides elimination from the structure Ti-Al.
Table 2. Explanation of the X-ray diffraction pattern shown in Fig. 3
№ |
Ti |
Al |
Θ |
d (hkl) |
2 Θ |
Al 3 Ti |
Al 2 Ti |
||
hkl |
d(hkl) |
hkl |
d(hkl) |
||||||
1 |
100* |
2.56295 |
11.55 |
3.8476 |
35.1 |
3.80 |
3.94 |
||
2 |
002 |
2.35004 |
19.15 |
2.3485 |
38.3 |
1.9 |
1.97 |
||
3 |
101 |
2.24952 |
20 |
2.2523 |
40 |
2.714 |
2.814 |
||
4 |
200 |
2.03508 |
22.3 |
2.03004 |
44.6 |
1.9 |
1.97 |
||
5 |
102 |
1.73046 |
26.45 |
1.7295 |
52.9 |
1.696 |
1.758 |
||
6 |
110 |
1.47797 |
31.55 |
1.4721 |
63.1 |
2.714 |
2.814 |
||
7 |
220 |
1.43874 |
32.4 |
1.4375 |
64.8 |
1.342 |
1.392 |
||
8 |
112 |
1.25124 |
38.1 |
1.2484 |
76.2 |
1.551 |
1.608 |
||
9 |
201 |
1.22665 |
38.9 |
1.2267 |
77.8 |
1.696 |
1.758 |
||
10 |
1.17427 |
222 |
41 |
1.1662 |
82 |
1.098 |
1.138 |
||
11 |
0.99014 |
400 |
1.01675 |
49.25 |
1.0168 |
98.5 |
0.95 |
0.985 |
|
12 |
331 |
0.93301 |
56.5 |
0.9241 |
111.3 |
0.871 |
0.903 |
||
13 |
420 |
0.90941 |
58.15 |
0.9069 |
116.3 |
0.850 |
0.881 |
Table 3. Comparison of Al2Ti phases, based on data of Tables 1 and 2
Al 2 Ti |
||||
№ |
hkl |
Table 1 |
hkl |
Table 2 |
1 |
100 |
3.94 |
100* |
3.94 |
2 |
111 |
2.277 |
002 |
1.97 |
3 |
101 |
2.814 |
101 |
2.814 |
4 |
200 |
1.97 |
200 |
1.97 |
5 |
102 |
1.758 |
102 |
1.758 |
6 |
220 |
1.392 |
110 |
2.814 |
7 |
112 |
1.608 |
220 |
1.392 |
8 |
311 |
1.187 |
112 |
1.608 |
9 |
222 |
1.138 |
201 |
1.758 |
10 |
400 |
0.985 |
222 |
1.138 |
11 |
331 |
0.903 |
400 |
0.985 |
12 |
420 |
0.881 |
331 |
0.903 |
13 |
420 |
0.881 |
-
2) Shear deformation mechanism of the atomic groups displacement in mesoscopic spaces were suggested.
а
b
Fig. 4. Cluster model of the transition from the structure with the bcc lattice to the hcp lattice through the fcc phase
Список литературы Evolution of phase composition of composite materials at contact of titanium-aluminium obtained by welding explosion
- Павлюкова Д.В. Структура и механические свойства слоистых материалов на основе титана и алюминия, полученных по технологии сварки взрывом и дополнительной термической обработки: Автореф. дисс. …канд. техн. наук. Новосибирск: НГТУ, 2011. 19 с.
- Лесков М.Б., Абкарян А.К., Анфилофьев В.В., Шелепова С.Ю., Герт С., Квеглис Л.И. Фазовый состав композиционного материала в зоне контакта алюминий-титан. Барнаул; Красноярск, 2016. С. 81-86
- Коржов В.П., Карпов М.И. Структура и высокотемпературная прочность при изгибе многослойного композиционного материала из интерметаллидов титана с алюминием. Вестник Тамбовского университета, 2013, 18(4-2).
- Ghosh G., Asta M. First-principles calculation of structural energetics of Al-TM (TM = Ti, Zr, Hf) intermetallics. Acta Materialia 2005, 53, 3225-3252.
- Зубков Л.Б. Космический металл: Все о титане. Раздел ГРНТИ: Машиностроительные материалы. М.: Наука, 1987. с. 128.
- Bataev I.A., Bataev A.A., Malib V.I., Pavliukova D.V. Structural and mechanical properties of metallic-intermetallic laminate composites produced by explosive welding and annealing. Novosibirsk, State Technical University, 2011. 10 с.
- Mali V.I., Pavliukova D.V., Bataev I.A., Bataev A.A., Smirnov A.I., Yartsev P.S., Bazarkina V.V. Formation of the intermetallic layers in Ti-Al multilayer composites. Novosibirsk, State Technical University, 2011. 6 с.
- Орлова Ю.А., Джес А.В., Квеглис Л.И. Кластерная самоорганизация при мартенситных превращениях. ВКГУ им С. Аманжолова. Усть-Каменогорск, 2016. С. 202-208.
- Shuster J.C., Ipser H.Z. Metallfde 1990, 81. 389.