Моделирование эффективных электропроводящих свойств полимерных нанокомпозитов со случайным расположением частиц оксида графена
Автор: Ташкинов М.А., Добрыднева А.Д., Матвеенко В.П., Зильбершмидт В.В.
Статья в выпуске: 2, 2021 года.
Бесплатный доступ
В последнее время композиционные материалы находят всё большее применение в различных индустриальных отраслях: в авиационной, морской и автомобильной промышленности, строительстве и др. Для выявления внутренних повреждений в полимерных композиционных материалах, таких как растрескивание матрицы, расслоение и др., получили активное развитие методы, основанные на измерении электропроводимости композиционного материала, которые дают возможность контролировать состояние композита в течение всего срока службы. В качестве матриц в композиционных материалах зачастую используются полимеры. Однако почти всегда чистые полимеры являются диэлектриками. Добавление в них нанонаполнителей, например графена и его производных, успешно используется для создания проводящих композитов на основе изоляционных полимеров. На конечные свойства наномодифицированных композитов могут влиять многие факторы, в том числе тип и собственные свойства нанообъектов, их дисперсия в полимерной матрице, межфазные взаимодействия. Работа посвящена изучению и моделированию эффективных электропроводящих свойств представительных объемов наномасштабных композиционных материалов на основе полимерной матрицы с распределенными в ней частицами оксида графена. В частности, изучены методы оценки эффективных, электропроводящих свойств, построены конечно-элементные модели представительных объемов полимерных матриц с частицами оксида графена, исследовано влияние эффекта туннелирования и влияние ориентации включений на электропроводящие свойства материалов. Исследована возможность использования моделей резистивных датчиков деформации, работающих на принципе эффекта туннелирования. На основе конечно-элементного моделирования и инструментария теории графов созданы подходы для расчета изменения проводящих свойств представительных объемов наномодифицированной матрицы, подверженных механическому нагружению.
Композиционный материал, полимер, углеродные наночастицы оксид графена, эффективные свойства, электропроводность, представительный объем, перколяция, туннелирование, межфазные зоны
Короткий адрес: https://sciup.org/146282047
IDR: 146282047 | DOI: 10.15593/perm.mech/2021.2.15
Текст научной статьи Моделирование эффективных электропроводящих свойств полимерных нанокомпозитов со случайным расположением частиц оксида графена
ВЕСТНИК ПНИПУ. МЕХАНИКА № 2, 2021PNRPU MECHANICS BULLETIN
Одной из основ технического прогресса является эволюция материалов и их свойств. В последнее время композиционные материалы находят всё большее применение в различных индустриальных отраслях: в авиационной, морской и автомобильной промышленности, строительстве и др. Композиционные материалы состоят из двух и более армирующих элементов, обеспечивающих улучшенные физико-механические характеристики материала, и из основы (матрицы), которая связывает эти армирующие элементы.
Для выявления внутренних повреждений в полимерных композиционных материалах, таких как растрескивание матрицы, расслоение и др., в настоящее время широко применяются методы неразрушающего контроля, например, метод акустической эмиссии или ультразвуковой контроль [1–5]. Такие методы требуют специального оборудования для обнаружения повреждений, а также решения сопутствующих технических проблем. С целью преодоления этих трудностей активное развитие получили методы, основанные на измерении электропроводимости композиционного материала, которые дают возможность контролировать состояние композита в течение всего срока службы.
В качестве матриц в композиционных материалах зачастую используются полимеры, которые почти всегда являются диэлектриками. Таким образом, чтобы обнаружить ранние режимы разрушения, полимерная матрица должна стать электропроводящей [6–9]. Это может быть достигнуто за счет введения в матрицу проводящих наночастиц, которые обладают спектром физических свойств, намного превосходящих соответствующие свойства матрицы. Благодаря своим высоким механическим характеристикам, высокой электрической и тепловой проводимости углеродные нанонаполнители широко рассматриваются в качестве возможной стратегии улучшения механического и/или проводящего характера полимерных нанокомпозитов, что обычно приводит к получению конечных материалов с многофункциональными характеристиками.
Наночастицы на основе углерода могут быть классифицированы в зависимости от их структуры и размеров. Наиболее распространенными с точки зрения использования частицами являются углеродные нанотрубки (УНТ) и графен (рис. 1). УНТ, графен и их производные представляют собой материалы низкой плотности в наноразмерном масштабе, что делает их подходящими кандидатами для использования при изготовлении высокоэффективных функциональных полимерных композитов. Их геометрические характеристики обеспечивают возможность взаимодействия поверхности с полимерами, что приводит к значительному улучшению механических и/или проводящих свойств.
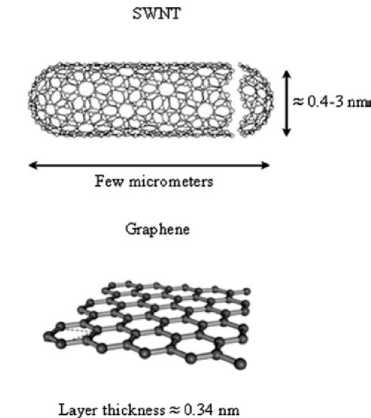
Рис. 1. Типы наночастиц на основе углерода: одностенные углеродные нанотрубки (SWNT), графен [10]
Fig. 1. Types of carbon-based nanoparticles: single-wall carbon nanotubes (SWNT), graphene [10]
Из всех углеродных наночастиц графен и его модификации остаются наиболее многообещающими кандидатами для создания электропроводящих полимерных композитов. Открытие графена произвело революцию в области наноматериалов, так как двумерные аллотропы углерода оказались способными проявлять особенные свойства, такие как высокая площадь поверхности, соотношение сторон, предел прочности при растяжении, электрическая и тепловая электропроводность, эффективность экранирования от электромагнитных помех, гибкость, прозрачность и низкий коэффициент теплового расширения [10, 11, 20–22, 12–19]. Графен может быть подходящей заменой УНТ с точки зрения практичности и стоимости. Его преимущества по сравнению с УНТ в том, что вязкость графеновых композитов значительно ниже при тех же нагрузках, а также в том, что большее количество наполнителя может быть внедрено в матрицу, что приводит к более высоким предельным значениям проводимости [23]. Помимо того, что графен является высокопроводящим материалом, он почти прозрачен и может поглощать около 2 % света, причем в широком интервале температур. В настоящее время существуют модификации, которые отличаются от чистого графена тем, что они могут содержать несколько уложенных друг на друга слоев (таких как графеновые нанопластинки) или иметь различные химические структуры (такие как оксид графена).
На конечные свойства наномодифицированных композитов могут влиять многие факторы, в том числе тип и собственные свойства нанообъектов, их дисперсия в полимерной матрице, межфазные взаимодействия [24, 25]. Электропроводность материала зависит от структуры создаваемой по всему нанокомпозиту проводящей сети путем достижения необходимой однородной дисперсии наночастиц в матрице, например, с использованием ультразвуковой обработки [26]. Другой метод повышения уровня дисперсии предполагает предварительную функционализацию наночастиц, которая является возможной стратегией повышения электропроводности нанокомпозитов на основе углерода при более низкой концентрации нанонаполнителя [27]. Некоторые приложения могут требовать преимущественной электрической проводимости материала в определенном направлении. Поэтому для распределения наночастиц в тонких пленках или в объемных матрицах используется ряд методов, в том числе методы выравнивания, индуцированные силой, магнитным и электрическим полями [28–31]. Тем не менее задача получения полной и однородной дисперсии отдельных графеновых частиц в композите остается нерешенной. Важную подготовительную роль играет предварительное моделирование физико-механических свойств полимерных нанокомпозитов и изучение характера их изменения при деформировании материалов.
Целью данной работы является изучение эффективных электропроводящих свойств представительных объемов композиционных материалов на основе полимерной матрицы со случайно распределенными в ней частицами оксида графена на основе конечноэлементного анализа трехмерных представительных объемов, а также изучение возможности создания сенсоров деформаций с использованием численных моделей расчета эффективных свойств электропроводности материалов при деформировании представительных объемов.
1. Моделирование проводимости наномодифицированных материалов с учетом сопротивления туннелирования
Для численной оценки электропроводности полимерных нанокомпозитов, содержащих различные типы углеродных наночастиц, от наиболее распространенных УНТ до материалов на основе графена и его модификаций, используется ряд методов, среди которых можно выделить численное моделирование, обработку изображений и аналитические модели [13, 15, 38–41, 19, 23, 32–37]. Большинство этих исследований направлено на исследование абсолютного значения электропроводности и/или минимизацию критической концентрации углеродных наночастиц, необходимой для достижения электропроводности. Критическая концентрация может быть определена на основе конкретных моделей проводимости, наиболее распространенной из которых явля- ется так называемая электрическая модель перколяции [27, 42], согласно которой электрическая проводимость σ нанокомпозита резко возрастает при увеличении концентрации наночастиц (порог перколяции, ϕ ). График такой зависимости принимает характерную S-образную форму, демонстрируя три явных состояния: изолирующий, перколяционный и проводящий.
ст = СТ о (Ф-Ф c ) e для ф>ф c (1)
Наиболее широко используемой моделью проводимости в полимерных нанокомпозитах является степенная модель, основанная на традиционной теории перколяции, которая согласуется с экспериментальными измерениями электропроводности. Перколяция и электропроводность оценивались путем распознавания соединительной сети. Ряд моделей был разработан в попытке качественно определить влияние расположения УНТ на электропроводность нанокомпозитов. В других моделях электропроводности нанокомпозитов углеродные наночастицы были рассмотрены в качестве случайных резисторов, рассеянных по всей изолирующей полимерной матрице. Они включают в себя двумерное, псевдо-трехмерное и трехмерное моделирование [24, 25].
Показано, что при низких концентрациях нанонаполнителей наилучшие результаты с точки зрения электропроводности часто достигаются за счет обеспечения сочетания правильной дисперсии ориентации и относительно плохого пространственного распределения нанонаполнителей, что обеспечивает физический контакт между наночастицами и образование проводящей сети по всему материалу (рис. 2).
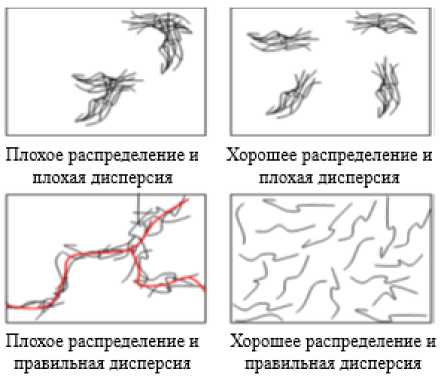
Рис. 2. Влияние распределения и дисперсии нанонаполнителя на электропроводность полимерных нанокомпозитов. Эффективный электрический путь гарантирован только тогда, когда нанонаполнитель плохо распределен и хорошо диспергирован в матрице (обозначен красным) [43]
Fig. 2. Influence of the distribution and dispersion of the nanofiller on the electrical conductivity of polymer nanocomposites. An efficient electrical path is only guaranteed when the nanofiller is poorly distributed and properly dispersed (indicated in red) [43]
При отсутствии контакта между проводящими наночастицами композит остается диэлектриком. Если частицы наполнителя образуют соединенную сеть, электроны могут перемещаться по этой сети, таким образом, композит становится проводящим. Существует также состояние, при котором частицы наполнителя не находятся в прямом контакте, а связаны посредством туннелирования электронов через границу между частицами. Туннельная проводимость представляет собой квантовый эффект прохождения электронов через матрицу между соседними проводящими частицами, находящимися на малом расстоянии друг от друга [38, 39, 44]. Толщина полимерной матрицы между частицами, обеспечивающая данный эффект, зависит от физических свойств компонент и представляет собой величину порядка нескольких нанометров. Эффект квантового туннелирования является важным элементом, обусловливающим механизм реализации электропроводности нанокомпозитов. Расстояние между частицами уменьшается с увеличением концентрации наполнителя, соответственно, возрастает роль туннельной проводимости.
Минимальная доля наполнителя, при которой система еще является проводящей, определяется порогом перколяции. Немногие существующие модели могут предсказать электропроводность в полном диапазоне концентраций наполнителя по обе стороны от порога перколяции. Большинство доступных моделей применимы для микрочастиц, но не отражают порог перколяции для низких концентраций мелкомасштабного наполнителя в виде наночастиц в полимерных композитах. Фактически, большинство этих моделей игнорируют внутреннее сопротивление частиц и туннелирование между контактирующими проводящими частицами и рассматривают все контактные сопротивления как равные. К тому же, зачастую модели проводимости нанокомпозитов не учитывают межфазные области вокруг наночастиц. Межфазные зоны обычно образуются в полимерных нанокомпозитах из-за значительной площади взаимодействия между полимерной матрицей и наполнителем в виде наночастиц [39]. Фактически, межфазная область может отличаться как от полимерной матрицы, так и от наночастиц, обладая промежуточными свойствами. Под толщиной межфазного слоя подразумевается расстояние между поверхностью наночастиц и объемной полимерной матрицей. Кроме того, межфазные области могут создавать непрерывные сети, которые снижают порог перколяции и могут создавать более крупные проводящие сети в нанокомпозитах.
Хотя классические перколяционные подходы широко использовались для моделирования электропроводности полимерных нанокомпозитов, содержащих проводящие наночастицы, во многих работах отмечалось, что они плохо соответствуют большинству экспериментальных результатов [26, 27, 45]. Были рассмотрены другие подходы, основанные на туннельной проводимости, такие как туннельная перколяция и двухэкспонентное феноменологическое уравнение перколяции, основанные на теории обобщенной эффективной среды, а также комбинированные модели [46]. В работах [34, 47] была рассмотрена важность существования несовершенной границы раздела между наночастицами и изолирующей матрицей, а также межфазного туннелирования с учетом вклада скачкообразного изменения потока электронов между наночастицами и использования статистической функции Коши для прогнозирования увеличения эффекта туннелирования вблизи порога перколяции для нанокомпозитов на основе графена.
Электрические свойства нанокомпозитов оцениваются путем анализа трех основных аспектов, влияющих на изменение общей проводимости нанокомпози-та/полимера:
-
1. Изменение сопротивления из-за проводящих сетей, образованных проводящими частицами, которые находятся в контакте между собой.
-
2. Сопротивление туннелирования для проводящих частиц, которые разделены матрицей.
-
3. Пьезорезистивность углеродных наночастиц.
Для модели сети резисторов, как показано на рис. 3, где схема резисторной модели со случайным распределением наночастиц на примере 2D-модели с двумя точками контакта i и j с соседними частицами, проводимость g между частицами i и j (обратная ве личина сопротивления R ) можно оценить как
S
Я = Ст —, gj ij
где / у - расстояние между точками i и j ; ст - электрическая проводимость наночастицы; S – площадь поперечного сечения наночастицы. Прямой контакт между частицами предполагается идеальными (с нулевым сопротивлением).
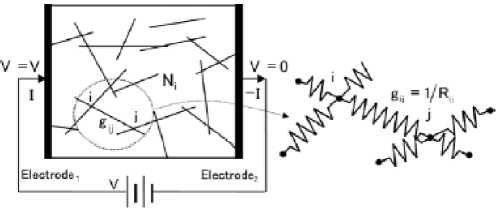
Рис. 3. Схема резисторной модели со случайным распределением наночастиц [38]
Fig. 3. Diagram of a resistor model with a random distribution of nanoparticles [38]
Любой перколяционный путь в представительном объеме можно представить как совокупность чередующихся резисторов, представляющих сопротивление наночастиц и туннельное сопротивление. Основываясь на известном матричном представлении для сети резисторов и действующем законе Кирхгофа, можно оценить полный ток I в системе при приложенном напряжении.
Макроскопическая электропроводность нанокомпозитов может быть затем оценена по закону Ома. Внутреннее сопротивление R вдоль углеродной наночастицы между двумя контактными узлами ( i , j ) оценивается как [44, 48]:
R j = px L& , П R
где р - удельное сопротивление; L и R длина и радиус частицы, соответственно.
Когда углеродные частицы хорошо диспергированы и их объемная доля в изолирующей полимерной матрице мала, отдельные наночастицы разделяются молекулами полимера, и из-за малого расстояния между соседними частицами необходимо исследовать возможный туннельный эффект и его влияние на электропроводность. С точки зрения морфологии случайное распределение и возможные пути туннелирования между соседними наночастицами можно экспериментально исследовать с помощью сканирующей электронной микроскопии (СЭМ). Туннельное сопротивление между двумя соседними наночастицами определяется зависимостью [38, 44, 49–51]
tunnel
V
AJ
h2d exp
Ae 2 V2 m " к
где J – плотность туннельного тока; V – разность электрических потенциалов; e – электрический заряд; m – масса электрона; h – постоянная Планка; d – расстояние между частицами; λ – высота барьера (для эпоксидной смолы – 0,5–2,5 эВ); A – площадь поперечного сечения туннеля (в качестве приближения часто используется площадь поперечного сечения наночастицы).
Определяющую роль туннельного сопротивления можно объяснить двумя следующими факторами: большой разницей сопротивления туннелирования и отдельных наночастиц, а также уникальной структурой резисторной сети, состоящей из чередования этих двух типов резисторов. Такая конфигурация эффективных сетей и факт, что сопротивление туннелирования намного больше по сравнению с внутренним сопротивлением наночастицы, оправдывают создание упрощенных моделей, учитывающих только сопротивление туннелирования при расчете свойств проводимости.
2. Определение эффективных электропроводящих свойств наномодифицированной полимерной матрицы
Возможность учета дисперсности наполнителя является ключевым компонентом методов моделирования проводимости представительных объемов наномодифи-цированных композитов. Влияние особенностей морфологии нанокомпозитов на основе полимерной матрицы и оксида графена на эффективные электропроводя- щие свойства представительных объемов материалов с учетом эффекта квантового туннелирования и без него может быть исследовано на основе конечно-элементных моделей. Одним из классических подходов является создание моделей со структурой типа «твердое ядро, мягкая оболочка». Твердое ядро принимает размеры физической частицы графена, а мягкая оболочка окружает его на несколько нанометров, обозначая расстояние, на котором возможно туннелирование электронов. Ядра не могут проникать друг в друга, но оболочки могут пересекаться. Таким образом, при расчете данной модели методом конечных элементов имитируется туннельный эффект. На основе подобных подходов были успешно смоделированы пороги перколяции в композитах на основе графена [34, 52], а также УНТ [44]. Данный подход используется в работе для моделирования в явном виде влияния морфологических особенностей распределения включений в представительном объеме (матрице) на эффективные свойства электропроводимости.
Геометрическая структура представительного объема состоит из объемной полимерной матрицы (куб с размерами 1,72 мкм) с частицами в виде оксида графена (цилиндры с радиусом 1 мкм и толщиной 0,0013 мкм). Распределение углов ориентации включений было задано по равномерному закону в пределах от 0 до 30 или 45 град. Центры включений распределяются случайным образом внутри представительного объема. На включения накладывалось условие непересечения, т.е. при наличии пересечения размер включений уменьшается вплоть до его исключения из модели.
Было создано два типа моделей – с оболочками, имитирующими туннелирование, и без них. На рис. 4
изображены модели геометрии представительного объема наномодифицированной частицами оксида графена полимерной матрицы с различной степенью разброса угла ориентации частиц.
Параметры конечно-элементной сетки отличались для моделей, содержащих включения с оболочками и без них. Так, для моделей без оболочек величина элементов варьировалась от 0,0005 до 0,05, с оболочками – от 0,0375 до 0,000075.
Для численных расчетов эффективных характеристик были взяты следующие модельные свойства [23]. Абсолютная толщина оболочки составляет 0,002 мкм. Проводимость матрицы равна G = 0,001 Ом–1, проводимость частиц оксида графена G = 200 Ом–1, проводимость оболочки G = 40 Ом–1 определена по формуле (4) и соответствует проводимости туннелирования на расстоянии от проводящей частицы, равном толщине оболочки (0,002 мкм).
Методом конечных элементов были получены эффективные свойства проводимости представительных объемов с различным количеством включений и разбросом угла ориентации от 0 до 30 град, с учетом эффекта туннелирования и без него. Сравнение значений полученных свойств представлено в табл. 1. Из результатов видно, что с помощью данного подхода можно получить оценочные значения эффективных свойств, в том числе с учетом эффекта туннелирования. При этом прослеживается закономерность влияния морфологии представительных объемов оказывает на эффективные свойства – разность в значениях обусловлена случайностью разброса включений и углов их ориентации.
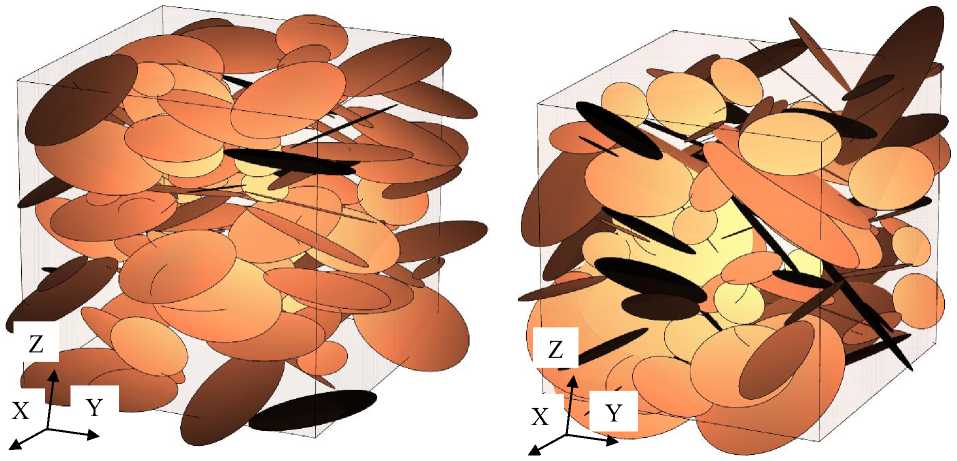
Рис. 4. Геометрия представительного объема с различным разбросом углов ориентации включений: а – равномерное распределение от 0 до 30 град; б – равномерное распределение от 0 до 45 град
Fig. 4. Geometry of a representative volume with different dispersions of inclusion orientation angles: ( a ) uniform distribution from 0 to 30 degrees; ( b ) uniform distribution from 0 to 45 degrees
Таблица 1
Проводимость нанокомпозитов по направлению X
Table 1
Conductivity of nanocomposites in X direction
Модель нанокомпозита |
Проводимость без оболочек ,Oм-1 |
Проводимость с оболочками, Ом-1 |
Модель с 25 включениями |
4.09 х 10 - 1 |
5.61 x 10 - 1 |
Модель с 20 включениями |
4.09 х 10 - 1 |
5.86 х 10 - 1 |
Модель с 24 включениями |
4.25 х 10 - 1 |
6.84 х 10 - 1 |
В табл. 2 приведено процентное соотношение, которое показывает, насколько влияние проводимости в направлении X отличается от проводимости в направлении Y . Видно, что в среднем проводимость по направлению Х на 79,1 % больше, чем в направлении Y , это говорит о том, что преимущественная ориентация частиц оксида графена существенно влияет на свойства проводимости.
Таблица 2
Процентное соотношение увеличения значений проводимости по направлению Y по сравнению с направлением X
Table 2
Percentage ratio of the increase of conductivity values in the Y direction compared to the X direction
Модель нанокомпозита |
Без оболочек, % |
C оболочками, % |
Модель с 25 включениями |
75,52 |
79,22 |
Модель с 20 включениями |
79,24 |
81,55 |
Модель с 24 включениями |
77,30 |
81,45 |
Проводимость представительных объемов без оболочек ниже, чем проводимость представительных объемов с оболочками, что говорит о вкладе эффекта туннелирования в значение эффективной проводимости. Этот факт можно использовать при создании моделей поведения резистивных датчиков деформации.
3. Численный анализ возможностей реализации деформационных сенсоров на основе полимерных нанокомпозитов
Резистивные датчики деформации – одна из основных областей применения эффектов электрического перколяционного поведения графеновых нанокомпозитов. Процесс деформирования материала вызывает изменение его микроструктуры и, следовательно, его электрического сопротивления. Электропроводность материала сильно зависит от структуры создаваемой проводящей сети по всему нанокомпозиту. Идеальная неоднородная структура на основе графена должна содержать непрерывные графеновые частицы с большим боковым размером, которые могут быть хорошо рас- пределены в матрице. Механизм изменения сопротивления представляет собой комбинацию двух процессов, оба из которых зависят от микроструктуры проводящей сети. Первое состоит в том, что по мере растяжения композита количество проводящих путей уменьшается по мере разрыва прямого контакта графеновых частиц (рис. 5) [23]. Второй механизм включает в себя отдаление проводящих частиц с одновременным уменьшением туннельной проводимости.
Учет эффекта туннелирования для перколяционной сети позволяет получить более приближенные к реальным значения проводимости в местах соединения наночастиц с матрицей. Это может быть использовано для того, чтобы создать стабильную электрическую сеть, которая, например, после начала разрушения материала могла бы сигнализировать о внутреннем повреждении композита.
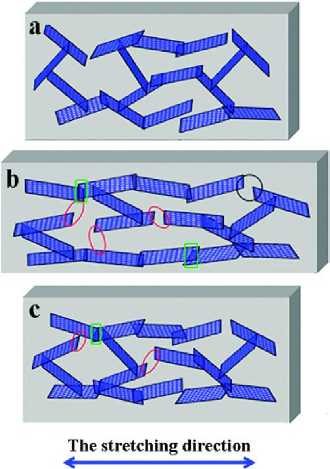
Рис . 5 . Влияние деформации на растягиваемый композит;
связность сети наполнителя разрывается (и иногда реформируется), изменяя проводимость матрицы [23]
-
Fig. 5. Effect of deformation on a tensiled composite; the filler bonds break (and sometimes reform), and change the matrix conductivity [23]
Процесс разрушения материала и соединений часто бывает обратимым, и поэтому нанокомпозиты на основе углеродных включений способны выполнять множество циклов деформации (например, 400 циклов с деформацией 60 % [23]) до разрушения. Лучшая производительность с большими деформациями возможна благодаря внедрению графена в гибкую матрицу.
Полученные в данной работе результаты демонстрируют принцип работы такого датчика. Предполагая, что модели без оболочки – это модели деформированного (растянутого) представительного объема, а модели с оболочкой соответствуют его исходному состоянию, можно зафиксировать, насколько изменяется сопротивление представительного объема при его деформирова- нии и нарушении перколяционных связей. Для демонстрации возможности использования изменения электрической проводимости представительного объема в качестве меры деформационного поведения материала может быть реализован более точный подход к оценке эффективной проводимости, описанный ниже.
В рамках концепции численного моделирования представительного объема наличие перколирующей проводящей сети может быть исследовано на основе измерения расстояний между проводящими включениями. Для этого в процессе пошагового создания представительных объемов для каждого нового включения решается задача расчета минимального расстояния попарно до всех других, уже присутствующих в модели, включений. Полученные расстояния впоследствии использовались в формуле (4) для расчета сопротивления туннелирования между отдельными частицами. При исследовании проводимости представительного объема по одному из ортогональных направлений рассчитывались все возможные пути в рамках присутствующей проводящей сети от одной грани к другой.
Один из наиболее удобных способов визуального представления перколяционных сетей в представительном объеме связан с использованием инструментария теории графов [13]. Вершины графа соответствуют включениям в полимерной матрице, при этом каждому включению присваивается свой номер. Если сопротивление, вычисленное по формуле (4) на основе расстояния между включениями, меньше сопротивления полимерной матрицы, вершины графа соединяются ребром. Исходя из физических свойств включений и полимерной матрицы может быть вычислено пороговое расстояние, при превышении которого эффект туннелирования не учитывается, что отражается в отсутствии связей между соответствующими вершинами в графе. Грани представительного объема, проводимость между которыми измеряется, также включаются в граф в виде вершин (как правило, первой и последней по порядковому номеру). Таким образом, представительному объему ставится в соответствие неориентированный граф, отражающий наличие перколяционных связей между находящимися в матрице включениями.
На основе полученного таким образом графа можно произвести расчет всевозможных путей соединения граней представительного объема через включения, образующие проводящую перколяционную сеть. Вычисление свойств электрической проводимости выполняется на основе теории электрических цепей. Электрическая цепь с последовательными и параллельными соединениями строится на основе составляющих графа: каждая вершина соответствует проводнику с сопротивлением, присущим проводящей частице, а каждое ребро соответствует проводнику с сопротивлением туннелирования, вычисленному исходя из соответствующего расстояния. Как было указано ранее, сопротивление отдельных частиц также может быть опущено, поскольку оно намного ниже сопротивления туннелирования. Общая проводимость представительного объема вычисляется путем расчета проводящих свойств электрической цепи.
Формирование перколяционной сети зависит от положения проводящих частиц в полимерной матрице. Проводимость исходной модели может быть оценена описанным выше методом на основе лишь геометрического анализа взаимного расположения элементов в матрице. Для оценки влияния деформации представительного объема на его проводящие свойства необходимо оценить изменение конфигурации перколяционной сети после деформирования и сравнить ее с конфигурацией исходной модели. Моделирование деформирования представительного объема может быть численно реализовано с использованием метода конечных элементов. Нагружение моделей происходит по тому же направлению, по которому происходит измерение сопротивления (проводимости).
Для построения графа деформированного представительного объема измеряются перемещения центров цилиндрических включений, для чего каждому центру ставится в соответствие определенный узел конечноэлементной сетки, перемещения которого фиксируются и измеряются после нагружения. Для смещенных таким образом включений заново анализируется перколяционная сеть и строится соответствующий ей граф. Вследствие деформации представительного объема расстояния между включениями изменяются, что отражается в изменении проводимости туннелирования (свойств проводимости ребер графа), а также в возможном исчезновении связей между определенными вершинами графа. Полученный граф показывает, какие связи были нарушены и существует ли до сих пор перколяция между гранями. Расчет проводимости электрической цепи, построенной на основе обновленного графа, позволяет получить сопротивление деформированного представительного объема. Сравнение данной величины с сопротивлением цепи исходного недеформированного представительного объема позволяет проанализировать, насколько увеличивается сопротивление при деформировании.
Ниже представлена численная реализация описанного подхода на примере полимерной матрицы с распределенными в ней проводящими частицами оксида графена. Были рассмотрены представительные объемы с разбросом углов ориентации включений в пределах от 0 до 30, а также от 0 до 45 град, по равномерному распределению. Примеры представительных объемов из каждой группы с пронумерованными включениями приведены на рис. 6. Исследовалась проводимость представительных объемов по направлению Y .
На рис. 7, 8 представлены графы взаимосвязи включений в перколяционной сети для двух представительных объемов. Как видно, в рассмотренных представительных объемах присутствовала только одна линия перколяции по данному направлению между гранями в плоскости X–Z . Грани представительного объема, между которыми измеряется проводимость, обозначены узлами с минимальным и максимальным номером.
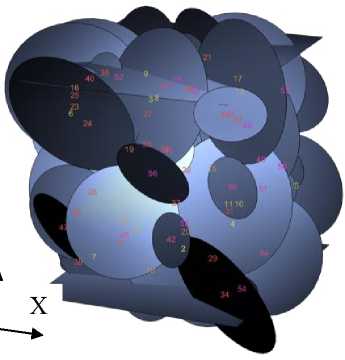
X
Y
X
w.
б
Рис. 6. Геометрические модели разброса включений оксида графена в представительных объемах полимерной матрицы в исходном недеформированном виде, с выделенными гранями, между которыми происходит измерение проводимости: а – разброс углов ориентации включений по равномерному закону распределения от 0 до 30 град; б – разброс углов ориентации включений по равномерному закону распределения от 0 до 45 град
-
Fig. 6. Geometric models of the scattering of graphene oxide inclusions in representative volumes of the polymer matrix in the original undeformed form, with selected faces, between which the conductivity is measured: ( a ) scattering of inclusion orientation angles according to the uniform distribution law from 0 to 30 degrees; ( b ) scattering of inclusion orientation angles according to the uniform distribution law from 0 to 45 degrees
Рис. 7. Граф недеформированного представительного объема с разбросом углов ориентации включений по равномерному закону распределения от 0 до 30 град
-
Fig. 7. Graph of an undeformed representative volume element with a scatter of the orientation angles of inclusions according to the uniform distribution law from 0 to 30 degrees
Рис. 8. Граф недеформированного представительного объема с разбросом углов ориентации включений по равномерному закону распределения от 0 до 45 град
-
Fig. 8. Graph of an undeformed representative volume element with a scatter of the orientation angles of inclusions according to the uniform distribution law from 0 to 45 degrees
Представительный объем подвергается одноосному растяжению по оси Y с перемещениями, равными 0,05 мкм. На рис. 9 показаны исходные позиции включений, входящих в перколяционную сеть, а также наложено измененное в результате деформации положение тех же включений. При этом за счет деформации включений и окружающей их матрицы в перколяционной сети происходят изменения, находящие отражение в графах взаимосвязи включений (рис. 10, 11).
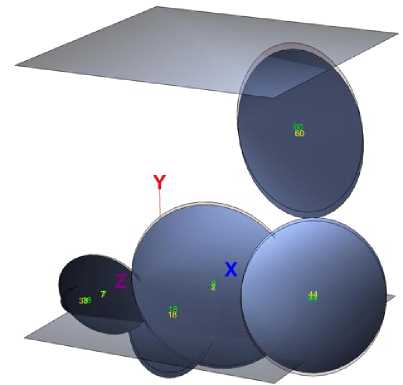
a
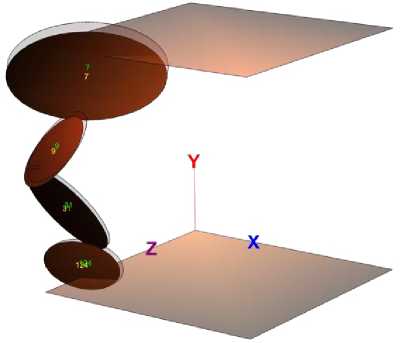
б
Рис. 9. Изменение положения включений, входящих в перколяционную сеть по направлению оси Y представительного объема, при деформации представительного объема в этом же направлении: а – разброс углов ориентации включений по равномерному закону распределения от 0 до 30 град; б – разброс углов ориентации включений по равномерному закону распределения от 0 до 45 град. Прозрачные пластины обозначают новое положение включений после растяжения представительного объема 0–45
Fig. 9. Change of the positions of the inclusions included in the percolation network in the direction of the Y axis of the representative volume element, when the representative volume element is deformed in the same direction: ( a ) a scatter of the inclusion orientation angles according to the uniform distribution law from 0 to 30 degrees; ( b ) a scatter of the inclusion orientation angles according to the uniform distribution law from 0 to 45 degrees. Transparent plates denote a new position of inclusions after stretching a representative volume element
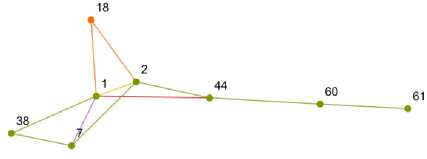
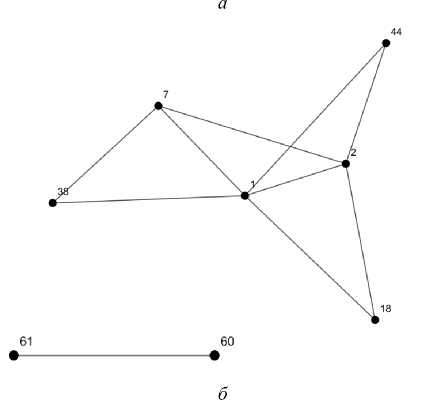
Рис. 10. Связность вершин графа, соответствующих включениям, входящим в перколяционную сеть представительного объема с разбросом углов ориентации включений по равномерному закону распределения от 0 до 30 град: а – до деформации;
б – после деформации
Fig. 10. Connectivity of the graph vertices corresponding to the inclusions included in the percolation network of the representative volume element with a scatter of inclusion orientation angles according to the uniform distribution law from 0 to 30 degrees: ( a ) before deformation; ( b ) after deformation
125 7 9 31 124 1
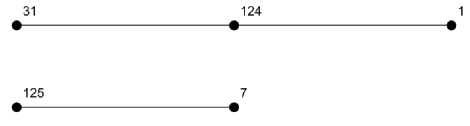
б
Рис. 11. Связность вершин графа, соответствующих включениям, входящим в перколяционную сеть представительного объема с разбросом углов ориентации включений по равномерному закону распределения от 0 до 45 град: а – до деформации;
б – после деформации
Fig. 11. Connectivity of graph vertices corresponding to inclusions included in the percolation network of the representative volume element with a scatter of the orientation angles of inclusions according to the distribution uniform law from 0 to 45 degrees: ( a ) before deformation; ( b ) after deformation
Из рис. 10 видно, что в процессе деформации цепь, благодаря которой в представительном объеме наблюдалась перколяция, разрушается. В частности, в первом случае (см. рис. 10, а) включение № 44 в результате растяжения представительного объема отдаляется от включения № 60 на расстояние, большее, чем пороговое значение расстояния туннелирования. Во втором случае прерывалась связь между включениями № 7 и № 9, а также между включением № 9 и № 31. В первом случае проводимость перколяционной сети до деформирования равна 3,3 Ом–1, во втором – 1,4 Ом–1. После деформирования проводимость цепей в пределах погрешности близка к проводимости полимерной матрицы. Эффект изменения проводимости продемонстрирован на примере представительных объемов с малым количеством включений, в котором образуется небольшое количество проводящих связей и, соответственно, небольшая перколяционная сеть. Нужно учитывать, что проводимость реальных образцов материала может существенно отличаться от проводимости отдельных перколяционных цепей, что требует уточнения количества перколяционных цепей на единицу объема материала и, как следствие, рассмотрения представительных объемов больших размеров.
Заключение
В работе изучены эффективные электропроводящие свойства представительных объемов композиционных материалов на основе полимерной матрицы с распределенными в ней частицами оксида графена. Рассмотрены влияние распределения и дисперсии наночастиц на электропроводность, принципиальные схемы распознавания перколяционных путей среди случайным образом распределенных наночастиц, моделирование туннель- ного эффекта в сети резисторов, влияние деформации на проводимость деформируемого материала. Получены результаты исследования влияния ориентации наночастиц на проводимость. Показано, что проводимость графеновых наночастиц существенно зависит от ориентации включений. С использованием моделей построения проводящих сетей на основе теории графов, а также с помощью построения моделей механики на основе метода конечных элементов показано, что благодаря эффекту туннелирования возможно создание высокоточных датчиков деформаций на основе нанокомпозитов. Полученные модели в дальнейшем могут быть использованы при исследовании возможностей реализации концепции резистивного датчика деформаций с учетом различных свойств полимерной матрицы и конфигурации проводящей сети, образованной проводящими наномасштабными включениями.
Список литературы Моделирование эффективных электропроводящих свойств полимерных нанокомпозитов со случайным расположением частиц оксида графена
- Structural health monitoring for composite materials / J. Cai [et al.] // Compos. their Appl. - 2012. - С. 37-58. DOI: 10.5772/48215
- Diamanti K., Soutis C. Structural health monitoring techniques for aircraft composite structures // Prog. Aerosp. Sci. -2010. - Vol. 46, no. 8. - P. 342-352. DOI: 10.1016/j.paerosci.2010.05.001
- Lau K.-T. Structural health monitoring for smart composites using embedded FBG sensor technology // Mater. Sci. Technol. - 2014. - Vol. 30, no. 13. - P. 1642-1654. DOI: 10.1179/1743284714Y.0000000608
- Micromechanics of nanocomposites / N. Stern [et al.] // Compr. Compos. Mater. II. - 2018. - Vol. 6-8. - P. 1-27. DOI: 10.1016/B978-0-12-803581-8.10250-4
- The use of intelligent computational tools for damage detection and identification with an emphasis on composites - A review / G.F. Gomes [et al.] // Compos. Struct. - 2018. - Vol. 196, no. April. - P. 44-54. DOI: 10.1016/j.compstruct.2018.05.002
- Tserpes K., Kora C. A multi-scale modeling approach for simulating crack sensing in polymer fibrous composites using electrically conductive carbon nanotube networks. Part I: Micro-scale analysis // Comput. Mater. Sci. - 2018. - Vol. 154, no. May. - P. 530-537. DOI: 10.1016/j.commatsci.2018.07.048
- Graphene/epoxy interleaves for delamination toughening and monitoring of crack damage in carbon fibre/epoxy composite laminates / X. Du [et al.] // Compos. Sci. Technol. - 2017. -Vol. 140. - P. 123-133. DOI: 10.1016/j.compscitech.2016.12.028
- Matos M.A.S., Tagarielli V.L., Pinho S.T. Simulation of the electromechanical properties of carbon nanotube polymer nanocomposites for strain sensing // Proc. Am. Soc. Compos. 31st Tech. Conf. ASC 2016. - 2016.
- Obitayo W., Liu T. A Review: Carbon Nanotube-Based Piezoresistive Strain Sensors // J. Sensors. - 2012. - Vol. 2012. -P. 1-15. DOI: 10.1155/2012/652438
- Abbasi H., Antunes M., Ignacio Velasco J. Recent advances in carbon-based polymer nanocomposites for electromagnetic interference shielding // Prog. Mater. Sci. - 2019. - Vol. 103, no. October 2017. - P. 319-373. DOI: 10.1016/j.pmatsci.2019.02.003
- Recent Progress in Graphene/Polymer Nanocomposites / X. Sun [et al.] // Adv. Mater. - 2020. - Vol. 2001105. - P. 1-28. DOI: 10.1002/adma.202001105
- Development of polymer composites using modified, high-structural integrity graphene platelets / J. Ma [et al.] // Compos. Sci. Technol. - 2014. - Vol. 91. - P. 82-90. DOI: 10.1016/j.compscitech.2013.11.017
- Matveenko V.P., Tashkinov M.A. Modeling the Influence of Structure Morphology on the Physical and Mechanical Properties of Nanocomposites Based on a Polymer Matrix and Graphene Oxide // Mech. Solids. - 2020. - Vol. 55, no. 3. - P. 316-323. DOI: 10.3103/S0025654420030097
- Papageorgiou D.G., Kinloch I.A., Young R.J. Mechanical properties of graphene and graphene-based nanocomposites // Prog. Mater. Sci. - 2017. - Vol. 90. - P. 75-127. DOI: 10.1016/j.pmatsci.2017.07.004
- Mutlay I., Tudoran L.B. Percolation behavior of electrically conductive graphene nanoplatelets/polymer nanocomposites: Theory and experiment // Fullerenes Nanotub. Carbon Nanostructures. - 2014. - Vol. 22, no. 5. - P. 413433. DOI: 10.1080/1536383X.2012.684186
- Graphene-based composite materials / S. Stankovich [et al.] // Nature. - 2006. - Vol. 442, no. 7100. - P. 282-286. DOI: 10.1038/nature04969
- Papageorgiou D.G., Kinloch I.A., Young R.J. Gra-phene/elastomer nanocomposites // Carbon. - 2015. - Vol. 95. -P. 460-484. DOI: 10.1016/j.carbon.2015.08.055
- Graphene-reinforced elastomeric nanocomposites: A review / B. Mensah [et al.] // Polym. Test. - 2018. - Vol. 68, no. April. - P. 160-184. DOI: 10.1016/j.polymertesting.2018.04.009
- Mechanical properties of graphene nanoplatelet/carbon fiber/epoxy hybrid composites: Multiscale modeling and experiments / C.M. Hadden [et al.] // Carbon N. Y. - 2015. -Vol. 95. - P. 100-112. DOI: 10.1016/j.carbon.2015.08.026
- Tang L., Zhao L., Guan L. Graphene/Polymer Composite Materials: Processing, Properties and Applications // Advanced Composite Materials: Properties and Applications. January 31, 2017. - P. 349-419. DOI: 10.1515/9783110574432-007
- Graphene-based polymer nanocomposites / J.R. Potts [et al.] // Polymer (Guildf). - 2011. - Vol. 52, no. 1. - P. 5-25. DOI: 10.1016/j.polymer.2010.11.042
- Kumar A., Sharma K., Dixit A.R. A review of the mechanical and thermal properties of graphene and its hybrid polymer nanocomposites for structural applications // J. Mater. Sci. - 2019. - Vol. 54, no. 8. - P. 5992-6026. DOI: 10.1007/s10853-018-03244-3
- Electrical percolation in graphene-polymer composites / A.J. Marsden [et al.] // 2D Mater. - 2018. - Vol. 5, no. 3. DOI: 10.1088/2053-1583/aac055
- Simulations and electrical conductivity of percolated networks of finite rods with various degrees of axial alignment / S.I. White [et al.] // Phys. Rev. B - Condens. Matter Mater. Phys. - 2009. DOI: 10.1103/PhysRevB.79.024301
- Du F., Fischer J.E., Winey K.I. Effect of nanotube alignment on percolation conductivity in carbon nanotube/polymer composites // Phys. Rev. B - Condens. Matter Mater. Phys. -2005. DOI: 10.1103/PhysRevB.72.121404
- Electrical conductivity phenomena in an epoxy resin-carbon-based materials composite / M. Castellino [et al.] // Compos. Part A Appl. Sci. Manuf. - 2014. DOI: 10.1016/j.compositesa.2014.02.012
- The viability and limitations of percolation theory in modeling the electrical behavior of carbon nanotube-polymer composites / S. Xu [et al.] // Nanotechnology. - 2013. DOI: 10.1088/0957-4484/24/15/155706
- Improving the dispersion and integration of singlewalled carbon nanotubes in epoxy composites through functionalization / J. Zhu [et al.] // Nano Lett. - 2003. - Vol. 3, no. 8. - P. 1107-1113. DOI: 10.1021/nl0342489
- Xie X.L., Mai Y.W., Zhou X.P. Dispersion and alignment of carbon nanotubes in polymer matrix: A review // Mater. Sci. Eng. R Reports. - 2005. - Vol. 49, no. 4. - P. 89-112. DOI: 10.1016/j.mser.2005.04.002
- Bauhofer W., Kovacs J.Z. A review and analysis of electrical percolation in carbon nanotube polymer composites // Compos. Sci. Technol. - 2009. - Vol. 69, no. 10. - P. 1486-1498. DOI: 10.1016/j. compscitech.2008.06.018
- Customizing thermally-reduced graphene oxides for electrically conductive or mechanical reinforced epoxy nano-composites / J.M. Vazquez-Moreno [et al.] // Eur. Polym. J. - 2017. -Vol. 93, no. March. - P. 1-7. DOI: 10.1016/j.eurpolymj.2017.05.026
- Azoti W.L., Elmarakbi A. Multiscale modelling of graphene platelets-based nanocomposite materials // Compos. Struct. - 2017. - Vol. 168. - P. 313-321. DOI: 10.1016/j. compstruct.2017.02.022
- Effect of morphological state of graphene on mechanical properties of nanocomposites / O. Bayrak [et al.] // J. Mater. Sci. -2016. - Vol. 51, no. 8. - P. 4037-4046. DOI: 10.1007/s10853-016-9722-0
- Wang Y., Shan J.W., Weng G.J. Percolation threshold and electrical conductivity of graphene-based nanocomposites with filler agglomeration and interfacial tunneling // J. Appl. Phys. - 2015. - Vol. 118, no. 6. - P. 065101. DOI: 10.1063/1.4928293.
- Berhan L., Sastry A.M. Modeling percolation in high-aspect-ratio fiber systems. II. the effect of waviness on the percolation onset // Phys. Rev. E - Stat. Nonlinear, Soft Matter Phys. - 2007. - Vol. 75, no. 4. - P. 1-7. DOI: 10.1103/PhysRevE.75.041121
- Modeling electrical conductivities of nanocomposites with aligned carbon nanotubes / W.S. Bao [et al.] // Nanotechnology. - 2011. - Vol. 22, no. 48. - P. 485704. DOI: 10.1088/0957-4484/22/48/485704
- A morphological investigation of conductive networks in polymers loaded with carbon nanotubes / G. Lubineau [et al.] // Comput. Mater. Sci. - 2017. - Vol. 130. - P. 21-38. DOI: 10.1016/j.commatsci.2016.12.041
- Tunneling effect in a polymer/carbon nanotube nanocomposite strain sensor / N. Hu [et al.] // Acta Mater. -2008. - Vol. 56, no. 13. - P. 2929-2936. DOI: 10.1016/j.actamat.2008.02.030
- Zare Y., Garmabi H., Rhee K.Y. Roles of filler dimensions, interphase thickness, waviness, network fraction, and tunneling distance in tunneling conductivity of polymer CNT nanocomposites // Mater. Chem. Phys. - 2018. - Vol. 206. -P. 243-250. DOI: 10.1016/j.matchemphys.2017.11.017
- Multiscale electro-mechanical modeling of carbon nanotube composites / K. Grabowski [et al.] // Comput. Mater. Sci. - 2017. - Vol. 135. - P. 169-180. DOI: 10.1016/j.commatsci.2017.04.019
- Overview of polymer nanocomposites: Computer simulation understanding of physical properties / L. Wu [et al.] // Polymer (Guildf). - 2017. - Vol. 133. - P. 272-287. DOI: 10.1016/j.polymer.2017.10.035
- Balberg I., Sunshine S.A., Heaney M.B. Critical behavior of the electrical transport properties in a tunneling-percolation system // Phys. Rev. B - Condens. Matter Mater. Phys. - 1999. DOI: 10.1103/PhysRevB.59.12196
- Effects of the dispersion state and aspect ratio of carbon nanotubes on their electrical percolation threshold in a polymer / Bao H. Da [et al.] // J. Appl. Polym. Sci. - 2013. DOI: 10.1002/app.37554
- Yu Y., Song G., Sun L. Determinant role of tunneling resistance in electrical conductivity of polymer composites reinforced by well dispersed carbon nanotubes // J. Appl. Phys. -2010. - Vol. 108, no. 8. - P. 1-5. DOI: 10.1063/1.3499628
- Numerical investigation on the influence factors of the electrical properties of carbon nanotubes-filled composites / B. De Vivo [et al.] // J. Appl. Phys. - 2013. DOI: 10.1063/1.4811523
- Hansen N., Adams D.O., Fullwood D.T. Evaluation and development of electrical conductivity models for nickel nanostrand polymer composites // Polym. Eng. Sci. - 2015. DOI: 10.1002/pen.23914
- Hashemi R., Weng G.J. A theoretical treatment of graphene nanocomposites with percolation threshold, tunneling-assisted conductivity and microcapacitor effect in AC and DC electrical settings // Carbon N. Y. - 2016. - Vol. 96. - P. 474-490. DOI: 10.1016/j.carbon.2015.09.103
- Wang W., Jayatissa A.H. Computational and experimental study of electrical conductivity of graphene/poly(methyl methacrylate) nanocomposite using Monte Carlo method and percolation theory // Synth. Met. - 2015. -Vol. 204. - P. 141-147. DOI: 10.1016/j.synthmet.2015.03.022
- Effect of carbon nanotube geometry upon tunneling assisted electrical network in nanocomposites / W.S. Bao [et al.] // J. Appl. Phys. - 2013. - Vol. 113, no. 23. DOI: 10.1063/1.4809767
- Tunneling resistance and its effect on the electrical conductivity of carbon nanotube nanocomposites / W.S. Bao [et al.] // J. Appl. Phys. - 2012. - Vol. 111, no. 9. DOI: 10.1063/1.4716010
- Health monitoring for composite materials with high linear and sensitivity GnPs/epoxy flexible strain sensors / S. Lu [et al.] // Sensors Actuators, A Phys. - 2017. - Vol. 267. -P. 409-416. DOI: 10.1016/j.sna.2017.10.047
- Hicks J., Behnam A., Ural A. A computational study of tunneling-percolation electrical transport in graphene-based nanocomposites // Appl. Phys. Lett. - 2009. - Vol. 95, no. 21. -P. 1-4. DOI: 10.1063/1.3267079