Selective extraction of cobalt and copper from Chilean mine water by ion exchange resin
Автор: Abeywickrama J., Hoth N., Ussath M., Drebenstedt C.
Журнал: Горные науки и технологии @gornye-nauki-tekhnologii
Рубрика: Обогащение и переработка минерального и техногенного сырья
Статья в выпуске: 1 т.5, 2020 года.
Бесплатный доступ
Heavy metal-containing waste has become one of heavy environmental problems. Processing of such waste accumulated at waste dumps to extract valuable components is commercially beneficial activity and, in addition, decreases environmental impact. In this study, Lewatit MonoPlus TP 207 (TP 207) ion exchange resin was evaluated for its suitability for selective removal of cobalt (Co) and copper (Cu) from mine waste waters, generated by heap leaching waste materials at a Chilean copper mine. In series of laboratory scale fixed-bed ion exchange column tests, the effects of process parameters, such as waste water flow rate and regenerant (sulfuric acid) concentration, were studied in both waste water treatment by the ion exchange resin process and the resin regeneration (metals eluting) process. The regeneration process tests were performed using 1 % and 10 % sulfuric acid. The best separation of the metals (in the eluting process) was achieved with regeneration of TP 207 resin using 1 % sulfuric acid at flow rate of 1 bed volume per hour (BV/h).
Heavy metal-containing waste, ion exchange resin, fix-bed columns, adsorption/desorption, regenera-tion/elution, eluate, selective extraction
Короткий адрес: https://sciup.org/140250754
IDR: 140250754 | DOI: 10.17073/2500-0632-2020-1-25-29
Текст научной статьи Selective extraction of cobalt and copper from Chilean mine water by ion exchange resin
Copper is a metal of choice for technologists and important metal with wide range of industrial applications such as plumbing, electroplating, manufacture of wires/cables for various industries, building, machine building, etc. [5]. Chile is the main copper producer in the world, which satisfies more than 30 % of the world supply [4]. For the latest century, in El Salvador mining district, tailings were disposed not only around mine pits [3], but also over the shore [7]. This has resulted in formation of large tailings deposits in this area [9]. With time, these tailings have been exposed to atmosphere action that resulted in weathering, oxidation, and formation of 1–2 m thick low-pH (2.6–4) oxidation zone in upper layer of the tailings. Atmospheric leaching of the zone material results in leaching of bivalent metal cations, such as Cu2+, Ni2+, and Zn2+ in concentrations up to 2265 mg/l, 18.1 mg/l, and 20.3 mg/l, respectively, in the mine tailings drainage waters [7]. This is very dangerous situation because these heavy metal concentrations are much above the approved maximum concentration limits.
During past decades, many methods have been implemented to remove heavy metals from the drainage waters. Among many classical and modern techniques, ion exchange method has unique advantages such as high metal recovery rate, ion selectivity, lesser slurry generation, the meeting strict discharge environmental specifications, etc [1]. Modern ion exchange resins are produced from synthetic polymer substances such as styrene-divinyl-benzene copolymers [6]. Synthetic ion exchange resins have relatively large pores, that facilitates the exchange process [8].
Thus, the purpose of this study is to test modern ion-selective ion exchange resins for selective removal and separation of heavy metals from mine drainage water generated fro m Chilean
2020;5(1):25-29
МИСиС
heap leaching residuals. Correct selective extraction of these metals may be profitable for the mine due to minimizing remediation costs and decreasing environmental impact (and, thus, environmental payments/fines). Among other valuable metals in these leaching residuals, cobalt should be highlighted, whose price has significantly increased over the latest several years [2]. This study will be specially focused on separation of cobalt from copper when extracting from the mine drainage waters.
2. Experimental research
The material used to generate mine water in this study was collected from heap-leached stockpile at a Chilean copper open pit. This material was mixed with double-distilled water, and the mixture was stirred several times for three days to produce final water for the testing. The ion exchange column unit comprised polyvinylchloride (PVC) column 2.5 cm in diameter and 12 cm high connected with peristaltic pump. A bed volume (BV) was 10 ml and it was injected into the material of initial wet ion exchange resin loaded into the column. All fittings and pipework were made of polyethylene. The mine drainage water was pumped into the column in up-flow direction by the peristaltic pump at controlled flow rates (1 BV/h = 10 ml/h; 6 BV/h = 60 ml/h). The water was sampled at the column outlet at regular time intervals and was analyzed for cations using MP-AES method. After the water treatment (adsorption) cycle, the columns were regenerated (for eluting the metals) by either 1 or 10 % (w/w) sulfuric acid solution. These concentration ranges were chosen based on the resin product specification provided by LANXESS. The backwash and regeneration were performed by sulfuric acid solution, which was pumped from bottom to top into the column at flow rate of either 1 BV/h or 6 BV/h.
-
3. Findings and Discussion
-
3.1 . Tests on metal sorption on TP 207 ion exchange resin column
-
Fig.1, a shows the curves of copper and cobalt concentrations in contact with TP 207 resin at flow rate of 10 ml/h (1 BV/h). Initial increasing of cobalt concentration was observed at around 70 BV and achieved peak value of 28.8 mg/l at 137 BV. Then the concentration of cobalt decreased and reached the initial concentration at 228 BV. Thereafter the concentration fluctuated around the initial value. Cobalt was adsorbed between 0 – 70 BV and then desorbed until the end of the process. The test continued overnight. Therefore, no samples were taken in interval between 85 ‒ 130 bed volumes. Copper concentration growth started at 160 BV and reached peak at 225 BV. Afterwards copper concentration fluctuated around the initial level. This test lasted 12 days to reach completing copper sorption. Fig. 1, b shows similar curves for copper and cobalt concentrations in contact with TP 207 ion exchange resin at flow rate of 60 ml/h (6 BV/h). The test continued overnight, and no samples were taken in interval between 48 – 129 BV. Initial increasing of cobalt concentration was observed at about 45 BV, and peak value was achieved at around 115 BV. After 129 BV cobalt was desorbed from the resin. After 195 BV the flow was stopped for twelve hours. After the interruption (at 197 BV), significantly higher cobalt concentration (32.7 mg/l) was observed. Copper concentration growth started at about 115 BV, and then copper was desorbed from the column up to reaching peak concentration at 225 ‒ 240 BV. Notice that during the flow interruption for twelve hours at about 197 BV, copper concentration dropped from 101.7 mg/l to 49.8 mg/l. The resin could adsorb 417.5 mg of copper during the test process.
2020;5(1):25-29
МИСиС
a b
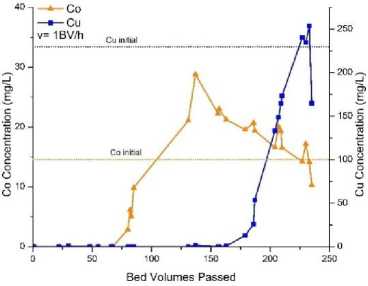
Fig. 1. Curves of cobalt and copper concentrations in contact with TP 207 ion exchange resin: a ‒ flow rate = 10 ml/h (1 BV/h); b ‒ flow rate 60 ml/h (6 BV/h)
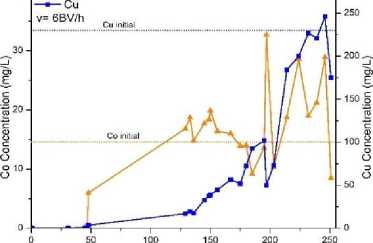
Bed Volumes Passed
3.2 Column TP 207 ion exchange resin regeneration (metal elution) tests
Fig. 2, a shows the column TP 207 ion exchange resin regeneration (using metal leaching (eluting) by 10 % sulfuric acid at flow rate of 1 BV/h) tests curves of copper and cobalt concentrations (for the resin taken after 120 BV). Intensive eluting both copper and cobalt started at 1 BV. Cobalt peak concentration of 533 mg/l was achieved at about 1.5 BV, which was the highest cobalt concentration achieved throughout this study. Copper peak concentration of 12,345 mg/l was achieved at about 2 BV. After that concentrations of both ions drastically decreased. At 2.5 BV the copper and cobalt concentration decreased to 4,903 mg/l and 60.2 mg/l, respectively. By that time, few small air bubbles near the bottom of the column were observed. Moreover, one side of the column was poorly regenerated. Starting from 3 BV, the copper and cobalt concentrations decline continued, but at lesser gradient. The copper concentration dropped below 50 mg/l after 7 BV. The cobalt concentration dropped below 15 mg/l (below detection limit of the MP-AES) after 5 BV. The tests recovered 309 mg of copper and 7.17 mg of cobalt during the resin regeneration (metal leaching) process. About 88% of the adsorbed cobalt were eluted within interval 0-3 BV. At the same time, both copper and cobalt were eluted in the same range of bed volumes. Therefore, no metal selection was achieved during the regener-ation/leaching (eluting) tests.
Fig. 2, b shows the column TP 207 ion exchange resin regeneration (using metal leaching by 1 % sulfuric acid at flow rate of 1BV/h) tests curves of copper and cobalt concentrations (for the resin taken after 120 BV). Intensive eluting cobalt started at 1 BV. Cobalt peak concentration of 328 mg/l was achieved at about 2 BV. Then the concentration dropped drastically to 47.6 mg/l at 4 BV. At 4 BV the regeneration process was interrupted for 12 hours. At 5 BV the cobalt concentration slightly increased, up to 62 mg/l and then dropped again to below 15 mg/l at 6 BV.
Intensive eluting copper started at 2 BV, and peak copper concentration of 4,132 mg/l was achieved at about 4 BV. Then the copper concentration drastically decreased to 2160 mg/l by 5 BV. Then, after the process interruption at 4 BV, the elution nature changed. The copper concentration started to grow again and achieved peak value of 3,247 mg/l at 7 BV. Thereafter the concentration gradually decreased and to below 100 mg/l after 16 BV. About 76.6 % of the adsorbed copper (288 mg) and 56 % of the adsorbed cobalt (7 mg) were eluted (leached) from the resin. Thus, the metals concentration curves at 1 % sulfuric acid showed marked selective elution of cobalt and copper.
2020;5(1):25-29
МИСиС
a
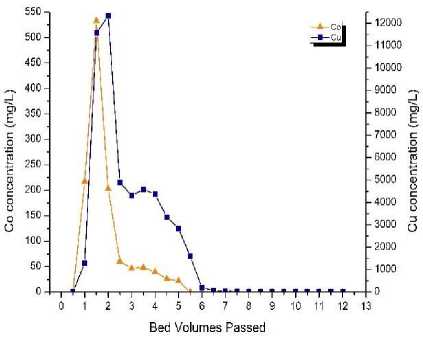
b
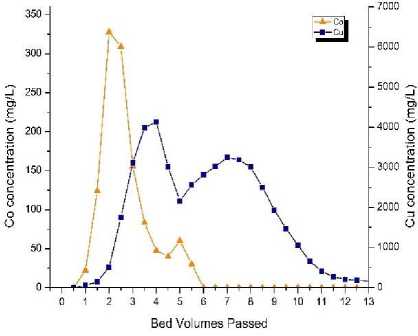
Fig. 2. Column TP 207 ion exchange resin regeneration tests – cobalt
and copper eluting (leaching) curves:
a ‒ by 10 % sulfuric acid; b ‒ by 1 % sulfuric acid
4. Conclusion
The performed tests and analysis showed that the Lewatit MonoPlus TP 207 (amino-di-acetic) ion exchange resin is suitable for selective extraction of copper and cobalt from waste water drainage generated by heap leaching waste material at the Chilean copper mine (open pit). Since the test feed solution (the waste water) has higher concentration of copper ions, it is very important to have higher amount of cobalt adsorbed by the resin during the water treatment process to achieve better elution as well as better separation of the metals in the resin regeneration (metal elution) process. Maximum adsorption of cobalt ions by the reins can be achieved by treatment the waste water until the column resin saturation (with cobalt) point. Further continuation of the treatment process results in desorbing the previously adsorbed cobalt ions due to the presence of better absorbable ions such as copper and zinc in the feed solution (the waste water). The treatment
References at higher flow rate (6 BV/h) results in earlier resin saturation with cobalt and its following greater desorption (as lesser absorbable ion). On the other hand, with the treatment at lower flow rate (1 BV/h) required more bed volumes before the resin saturation achieved and also resulted in sharp desorption curve. The best way for separation of copper and cobalt is using 1 % sulfuric acid as the resin regenerating solution (for eluting cobalt and copper). Increasing the sulfuric acid concentration lowers the elution process selectivity and, thus, the degree of the metals separation, as well as increases cobalt and copper concentrations in the eluate at lower flow rate (BV) values. Under the examined conditions, the tested ion exchange resin is capable to reduce the copper to cobalt ratio from 15 : 1 (feed waste water) to 8 : 1 during the resin regeneration process.
It is difficult to compare the results of this study with available literature data due to uniqueness of the studied mine waste water.
-
1. Abo-Farha S. A., Abdel-Aal A. Y., Ashour I. A., Garamon S. E. Removal of some heavy metal cations by synthetic resin purolite C100. Journal of hazardous materials . 2009;169(1-3):190-194.
-
2. Beardsmore T. J. Western Australia: a battery metal powerhouse . 2018.
-
3. Castilla J. C., Correa J. A. Copper tailing impacts in coastal ecosystems of northern Chile: from species to community responses. Moore M., Imray P., Dameron C., Callan P., Langley A., Mangas S. (eds.), Copper. National Environmental Health Forum Monographs, Metal Series . 1997;(3):81–92.
-
4. Correa J. A., Castilla J. C., Ramírez M., Varas M., Lagos N., Vergara S., Brown M. T. (1999). Copper, copper mine tailings and their effect on marine algae in northern Chile. In: Sixteenth International
2020;5(1):25-29
МИСиС
-
5. Das S. Batch study removal of copper ions from water by using ion exchange resin . Doctoral dissertation. 2014.
-
6. Demirbas A. Heavy metal adsorption onto agro-based waste materials: a review. Journal of hazardous materials . 2008;157(2-3):220-229.
-
7. Dold B. Element flows associated with marine shore mine tailings deposits. Environmental science & technology , 2006;40(3):752-758.
-
8. Elshazly A. H., Konsowa, A. H. Removal of nickel ions from wastewater using a cation-exchange resin in a batch-stirred tank reactor. Desalination . 2003;158(1-3):189-193.
-
9. Lee M. R., Correa J. A. An assessment of the impact of copper mine tailings disposal on meiofaunal assemblages using microcosm bioassays. Marine environmental research . 2007;64(1):1-20.
Seaweed Symposium . Springer, Dordrecht. P. 571-581
Список литературы Selective extraction of cobalt and copper from Chilean mine water by ion exchange resin
- Abo-Farha S. A., Abdel-Aal A. Y., Ashour I. A., Garamon S. E. (2009). Removal of some heavy metal cations by synthetic resin purolite C100. Journal of hazardous materials, 169(1-3), pp. 190-194.
- Beardsmore T. J. (2018). Western Australia: a battery metal powerhouse.
- Castilla J. C., Correa J. A. (1997). Copper tailing impacts in coastal ecosystems of northern Chile: from species to community responses. Moore M., Imray P., Dameron C., Callan P., Langley A., Mangas S. (eds.), Copper. National Environmental Health Forum Monographs, Metal Series No. 3:pp. 81-92.
- Correa J. A., Castilla J. C., Ramírez M., Varas M., Lagos N., Vergara S., Brown M. T. (1999). Copper, copper mine tailings and their effect on marine algae in northern Chile. In Sixteenth International Seaweed Symposium (pp. 571-581). Springer, Dordrecht.
- Das S. (2014). Batch study removal of copper ions from water by using ion exchange resin (Doctoral dissertation).
- Demirbas A. (2008). Heavy metal adsorption onto agro-based waste materials: a review. Journal of hazardous materials, 157(2-3), pp. 220-229.
- Dold B. (2006). Element flows associated with marine shore mine tailings deposits. Environmental science & technology, 40(3), pp. 752-758.
- Elshazly A. H., Konsowa, A. H. (2003). Removal of nickel ions from wastewater using a cation-exchange resin in a batch-stirred tank reactor. Desalination, 158(1-3), pp. 189-193.
- Lee M. R., Correa J. A. (2007). An assessment of the impact of copper mine tailings disposal on meiofaunal assemblages using microcosm bioassays. Marine environmental research, 64(1), pp. 1-20.