Surface activity of concrete waste powders
Автор: Ayzenshtadt A.M., Drozdyuk T.A., Danilov V.E., Frolova M.A., Garamov G.A.
Журнал: Nanotechnologies in Construction: A Scientific Internet-Journal @nanobuild-en
Рубрика: Manufacturing technology for building materials and products
Статья в выпуске: 2 Vol.13, 2021 года.
Бесплатный доступ
Introduction. One of the most large-tonnage wastes of the construction industry is a concrete scrap, obtained as a result of the dismantling of buildings and structures, the service life of which has reached its maximum or requires significant capital changes. In our opinion, works on the use of the resulting dust-like fraction are of particular interest. One of the promising methodological approaches in this direction is the observance of the principles of nanotechnology, which consists of obtaining finely dispersed components as active components of the created compositions. Materials and methods. Therefore, the objects of research in this work were fine powders obtained by mechanical grinding of lightweight (sample 1) and heavy (sample 2) concrete of a five-story residential panel house built in 1979. The elemental composition and specific surface area of the samples were determined, thermogravimetry of the studied powder systems was carried out, the surface tension of the experimental samples and the dispersion and polarization components of this indicator were determined by the OVRK method. A new methodological approach to the determination of the surface tension of powder systems is proposed, based on the determination of the functional dependence of the surface tension on the pressing force of the prototypes. The possibility of calculating macroenergy characteristics (atomization energy, specific mass atomization energy) of waste concrete samples is shown. These physicochemical indicators characterize the potential supply of internal energy of the system, which is capable of transforming into free surface energy during mechanical destruction of the material. Results and discussions. Thermogravimetric analysis of experimental concrete samples showed the presence of a residual amount of belite. Calculations of the surface activity of the samples showed that it is more preferable to use a powder obtained by crushing a sample of heavy concrete as an active additive in compositions capable of exhibiting the properties of a binding agent. Conclusions. To assess the activity of dusty fractions of concrete scrap as a component in binding compositions of the hydration type of hardening, it is proposed to use the value of the surface activity of powder systems as a criterion. The absolute numeric value of this criterion is equal to the ratio of the values of the free surface energy of the investigated powder and the specific mass energy of atomization of the initial waste concrete. It was found that for concrete waste the value of this criterion is determined by the polarization component of surface tension.
Recycled concrete, surface activity, composite binder, atomization energy, dust fraction of concrete scrap, thermogravimetric analysis
Короткий адрес: https://sciup.org/142226920
IDR: 142226920 | DOI: 10.15828/2075-8545-2021-13-2-108-116
Текст научной статьи Surface activity of concrete waste powders
The problem of processing and reuse of industrial waste is a key global factor in improving the ecological state of the environment. This provision fully applies to the building materials industry. In addition, research in this direction also allows not only to conserve natural resources, but also to significantly reduce the cost of final building materials. One of the most large-tonnage wastes of the construction industry is a concrete scrap, obtained as a result of the dismantling of buildings and structures, the service life of which has reached its maximum. Therefore, the significant interest of research aimed at solving the problem of using various fractions of concrete scrap in building materials science is understandable [1–4].
Of particular interest are studies on the use of the resulting dust fraction. For example, the results of studies [2, 5] on the effect of the dusty part of the screenings
MANUFACTURING TECHNOLOGY FOR BUILDING MATERIALS AND PRODUCTS of concrete scrap crushing when creating composite binders (CB) on the strength of cement stone showed that the strength of cement stone does not decrease significantly when secondary concrete dust is added to the cement. Thus, this screening exhibits the properties of a low-grade cement. However, a number of unresolved issues related to the determination of the activity of this fraction as a component of CB hinder its widespread use. This is largely due to significant time and labor-intensive costs in the traditional approach to solving this problem. At the same time, considering the pulverized fraction of concrete waste as a finely dispersed system consisting of micro- and nanosized particles with an increased energy potential, a nanotechnological approach can be applied to assess its ability to transformations. Thus, in [6–9], to determine the surface activity (kS) of such systems, a quantitative criterion is proposed, which is calculated based on the ratio of the values of the free surface energy of a highly dispersed system and the potential energy characteristic of its macrostate.
THEORETICAL FRAMEWORK
At present, we have developed a mechanism for the experimental determination of kS, which is numerically equal to the ratio of two characteristics: ES/Em. In this expression, the numerator is the free surface energy of the dispersed system (J/kg), and the denominator is the specific mass atomization energy (J/kg) of the test material. In turn, the calculation of the values of the free surface energy is carried out on the basis of the experimentally determined values of the specific surface of the investigated fine powder (Ssp, m2/kg) and its surface tension (σ, J/m2) [10–12] is equal to:
E S = σ• S уд . (1)
If there are practically no problems with the choice of the method for determining the specific surface area of highly dispersed powders (accurate and reproducible results are obtained by methods based on the BET theory and implemented by cryogenic adsorption of nitrogen on analytical systems Autosorb [13–15]), then the determination of surface tension (specific surface energy) of powder systems, for example, by the OVRK (Ouns, Wendt, Rabel and Kjellble) method [10, 11] requires the use of exact recommendations worked out for such standard fluids as decane, glycerin, water [16].
The specific mass energy of atomization is the algebraic sum of the standard enthalpies of formation of chemical compounds (atomization energy, Ea, J/mol) that make up the prototype taking into account their molecular (formula) masses (M, mol/kg) [17, 18]:
E m = E a / M . (2)
Standard enthalpies of formation of basic chemical compounds (in terms of their oxides) are presented in the corresponding reference literature [19–22]. Therefore, it seemed interesting to carry out a comparative analysis of the surface activity of the test samples of the dust-like fraction of concrete waste obtained after preliminary grinding of macro-samples to a specific surface close to that of a hydraulic cement (300 m2/kg). These values of the specific surface area of Portland cement are determined by the Kozeny-Karman gas permeability method using an installation, for example, PSH-10a.
In addition, the results of thermogravimetric analysis (TGA) of prototypes can represent significant results in terms of the scientific substantiation of the validity of the criterion used. This fact can be associated with the determination of the residual content of non-hydrolyzed calcium silicates during concrete hardening [5, 23, 24, 25]. These minerals, by mechanoactivation of the analyzed samples to a sufficient degree of dispersion, pass into an active state. For example, the degree of hydration of clinker minerals can be judged by the amount of weight loss (TGA curve) in the temperature range 400–500оC, which characterizes the loss of tightly bound water from the cement gel [26].
MATERIALS AND METHODS
In this work, the objects of study were concrete structures: internal wall panels and a floor slab (lightweight concrete, sample 1) and external wall panels (heavy concrete, sample 2) of a five-story residential panel house built in 1979 in the village of Obozersky, Arkhangelsk region. The residual strength of concrete samples was determined by the shock pulse method according to GOST 22690-2015 using an IPS-MG4 device. Before preliminary crushing, concrete samples were brought to constant weight in a drying oven and subjected to the procedure for measuring the true density by a standard method. Then the prototypes were split into pieces no more than 120 mm in size, from which the reinforcement was removed. Then the concrete fragments were crushed on a jaw crusher. The screenings were divided into fractions on a standard set of sieves. The elemental mineral composition of the obtained dust-like fractions was determined by X-ray fluorescence analysis using a “MetExpert” device. The specific surface area of the obtained concrete powders was measured by nitrogen sorption using an AUTOSORB-iQ-MP specific surface area and pore size analyzer and Kozeny-Karman gas permeability using a PSKh-10a unit. Thermogravimetric analysis of experimental samples (simultaneous thermogravimetric and differential thermal analysis) was carried out on an SDT Q650 thermogravimetric analyzer (TA Instrument Inc., USA) in a nitrogen atmosphere (flow rate 50 ml/min) at a heating rate of 10оC/min. Samples weighing 11 mg
MANUFACTURING TECHNOLOGY FOR BUILDING MATERIALS AND PRODUCTS
Table 1
Values of surface tension of reference liquids [27]
RESULTS AND DISCUSSIONS
Determination of the residual strength of test concrete samples showed that lightweight concrete (sample 1) can be attributed to class B22.5, and heavy (sample 2) – to B40. The average value of the true density (ρ) of the samples under study was 2253 kg/m3 for sample 1, and 2350 kg/m3 for sample 2. Grinding and fractionation of the initial concrete waste made it possible to obtain 9 size fractions each, differing in the size characteristics of the particles. For further research, we used fractions that had particle sizes of less than 0.14 mm. The elemental composition of the obtained prototypes of highly dispersed powders of concrete waste and Portland cement is presented in Table 2. The standard enthalpies of the formation of these oxides and their molecular weights are also given here.
The data presented in Table 2 made it possible to calculate the macroenergy characteristics of the samples under study. Thus, for sample 1 atomization energy (Ea) equal to 1510 kJ/mol, for sample 2 – 1281 kJ/mol, as for Portland cement – 1237 kJ/mol. Specific mass atomization energy (Em) for concrete (samples 1 and 2) and
Table 2
Elemental composition of experimental samples (in terms of oxides), enthalpies of formation (∆H298) of oxides, atomization energy (Ea) and their molecular weights (M)
Oxide |
Content, % |
∆H298 • 10–3, kJ/kmol |
Еа, kJ/mol |
М, g/mol |
||
Sample 1 |
Sample 2 |
Portland cement |
||||
SiO2 |
36.68 |
16.73 |
8.98 |
–910.7 [22] |
1859 |
60.0 |
CaO |
51.39 |
76.63 |
80.99 |
–635.1 [21] |
1062 |
72.0 |
Fe 2 O 3 |
11.18 |
5.89 |
6.72 |
–823.4 [19] |
2398 |
143.6 |
TiO2 |
0.75 |
0.76 |
– |
–944.0 [20] |
1916 |
80.0 |
SO3 |
– |
– |
3.31 |
–438.4 [19] |
1463 |
80.0 |
MANUFACTURING TECHNOLOGY FOR BUILDING MATERIALS AND PRODUCTS
Portland cement take the following values: 4.3•103 kJ/kg, 3.6•103 kJ/kg and 3.5•103 kJ/kg, respectively. The specific surface area of the analyzed samples, determined by the nitrogen sorption method, was 5000±30 m2/kg for the pulverized fraction of lightweight concrete (sample 1), for the pulverized fraction of heavy concrete (sample 2) – 5150±50 m2/kg and 500±10 m2/kg for Portland cement. Determination of the specific surface area of prototypes on the PSKh-10a installation showed the following specific surface area: 260 m2/kg – for sample 1, 240 m2/kg – for sample 2. The underestimated results in terms of the specific surface area, obtained on the PSKh device, can be explained by the complex structure of the particles of the concrete test samples. Thus, gas filtration through a layer of powder in this method is carried out at a pressure close to atmospheric, in the mode of “leakage” of gas flows through the pores of the powder body. However, in this case, the developed surface of the powder particles is not taken into account (the viscous flow passes only along the outer surface of the powder particles). This effect is excluded when determining the specific surface area of dispersed systems by nitrogen sorption. However, the experiment carried out allows us to conclude that there is a developed pore structure of powder prototypes of concrete, in contrast to particles of Portland cement.
Taking into account the values of the true density of the analyzed objects and the specific surface area, the diameter of the equivalent sphere (as the dimensional characteristic of porous particles of cornerstone asymmetric shape) (deq) of particles was calculated according to the following expression [28]:
d eq = 2/ ρS sp . (3)
For samples 1 and 2, this parameter is 177 nm and 165 nm, respectively.
The results of thermogravimetric analysis of sample 1 and Portland cement are presented in Figures 1 and 2.
In the presented diagrams (Fig. 1), in our opinion, one should pay attention to the following characteristic temperature ranges. Thus, in the temperature range of 400–500оC, the sample weight loss is associated with the removal of strongly bound water from the cement gel. The total weight loss of the sample due to its temperature modification was 16%. In addition, there is a clear endothermic effects in the region of 570, 735 and 1300оC. In the first case, the manifested effect is associated with the modification transformation of quartz (α → β). Endothermic effects in the temperature ranges of 730–800оC and 1300–1350оC are associated with the presence of a residual (not consumed in the main process of hydration) amount of dicalcium silicate (2CaO•SiO2). The endothermic effects in the temperature ranges of 420–430 and 900–1200оC, accompanied by a loss of sample weight, can be associated with the decomposition of calcite (CaCO3) and the release of CO2.
Table 3 shows the primary experimental data of the experiments obtained by the OVRK method and the calculated values of the surface tensions of the prototypes.
To determine the value of the surface tension (σ0) corresponding to the initial powder system without its forced compaction, which is necessary in the implementation of experimental methods for determining
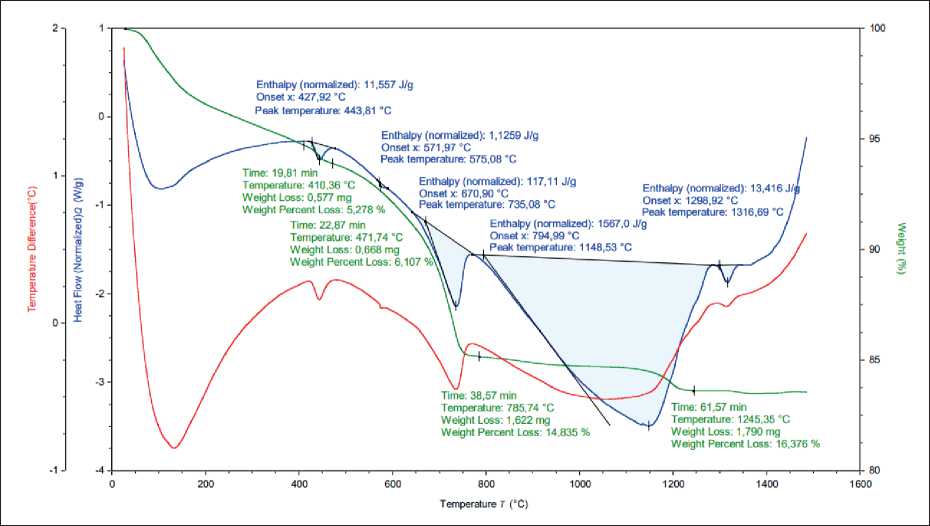
Fig. 1. TGA of the concrete sample
MANUFACTURING TECHNOLOGY FOR BUILDING MATERIALS AND PRODUCTS
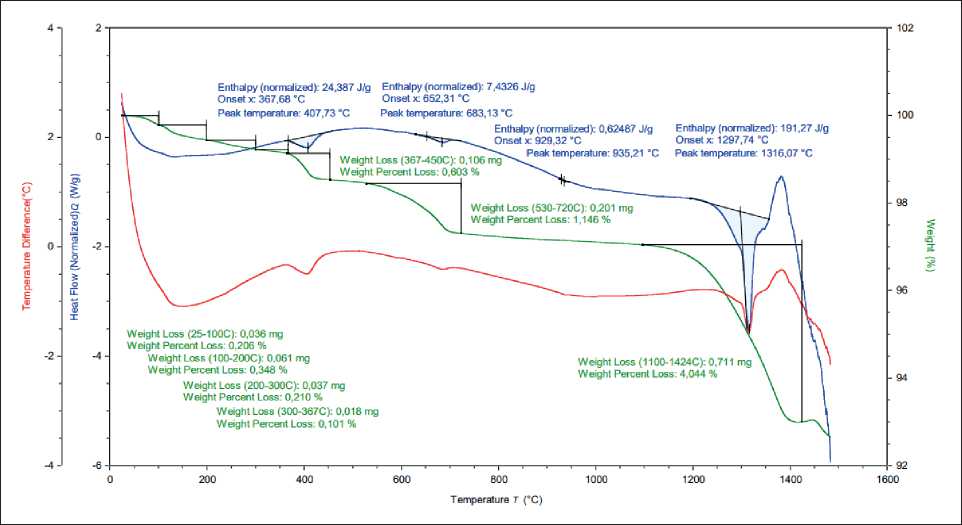
Fig. 2. TGA of a Portland cement sample
Table 3
Contact angles of wetting of test powders and calculated values of surface tensions by the OVRK method
The surface tension of condensed dispersed systems is the specific surface energy, the value of which is determined by the van der Waals interaction of particles (dispersion component) and the presence of active surface centers (polarization component). Considering this provision, it should be noted that for concrete powders (samples 1 and 2) the polarization effect prevails over the dispersion interaction. However, for samples of heavy concrete, this difference is more significant than for light concrete. This may be due to the fact that dur-
MANUFACTURING TECHNOLOGY FOR BUILDING MATERIALS AND PRODUCTS
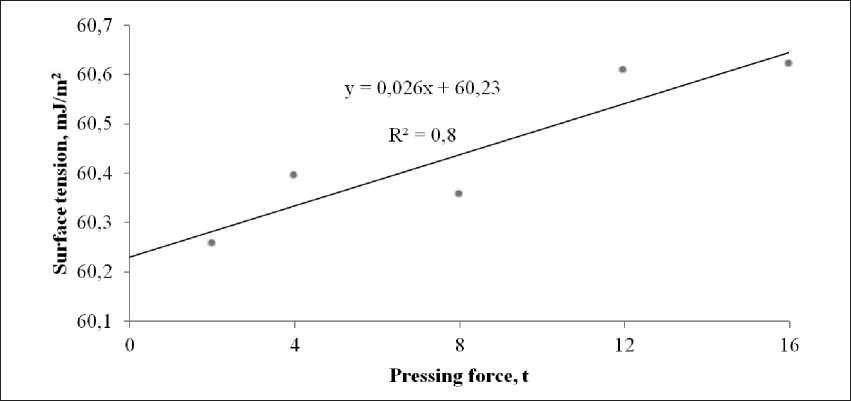
Fig. 3. Functional dependence σS = f (T) for lightweight concrete powder (sample 1)
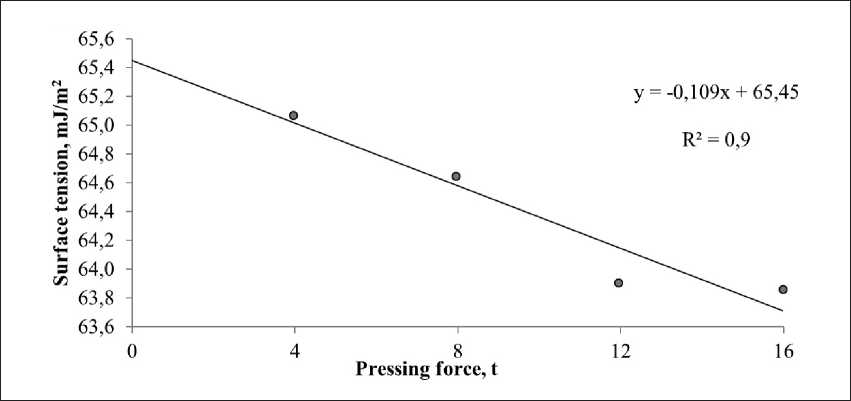
Fig. 4. Functional dependence σS = f (T) for lightweight concrete powder (sample 2)
ing the pressing process, additional destruction of the structure of the particles of these systems occurs. In our opinion, the presence of such an effect is associated with the anomalous value of the dispersion component of the surface tension at a force of 2 tons for sample 2, which is not compatible with the rest of the data set. Based on this fact, this experimental point for sample 2 was not used when approximating the functional dependence σS = f (T) by the equation of a straight line. In addition, the experimental results showed that an increase in the pressing force leads to a more significant increase in the van der Waals dispersion interaction of particles in lightweight concrete samples than in heavy concrete samples (Fig. 5). Thus, the slope of the functional dependence σsd = f (T) for sample 1 is more than two times higher than for sample 2. At the same time, the decrease in the values of the polarization components of the surface tension with an increase in the pressing force of the test samples occurs at almost the same speed (Fig. 6).
Physicochemical and energy characteristics of the surface of the test powders are presented in Table 4.
It can be noted that for the powder of light and heavy concrete, there is practically equality of the ratio of the surface tension component (σsd/σsp), varying in the range 0.5÷0.6, however, and the absolute value of the polarization component of the surface tension for sample 2 is higher than for sample 1. This fact indicates an increased content of active centers of the surface of the powder system of heavy concrete, which is the dominant factor in the manifestation of the greater reactivity of particles. Calculations of the surface activity of the samples showed that, as an active additive in compositions capable of exhibiting the properties of a binding agent, it is most preferable to use a powder obtained by crushing sample 2.
MANUFACTURING TECHNOLOGY FOR BUILDING MATERIALS AND PRODUCTS
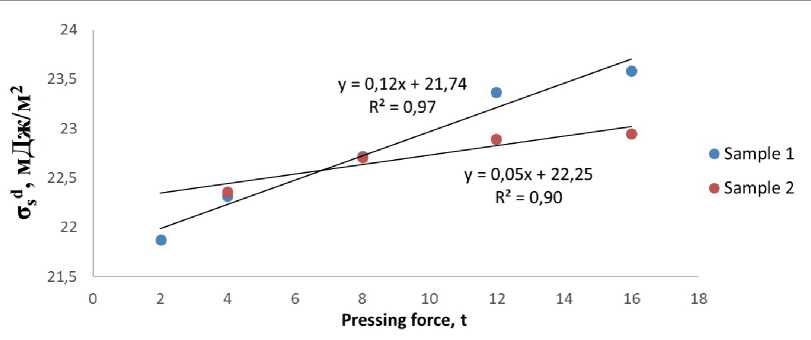
Fig. 5. Functional dependence of the form σsd = f (T) for concrete powders
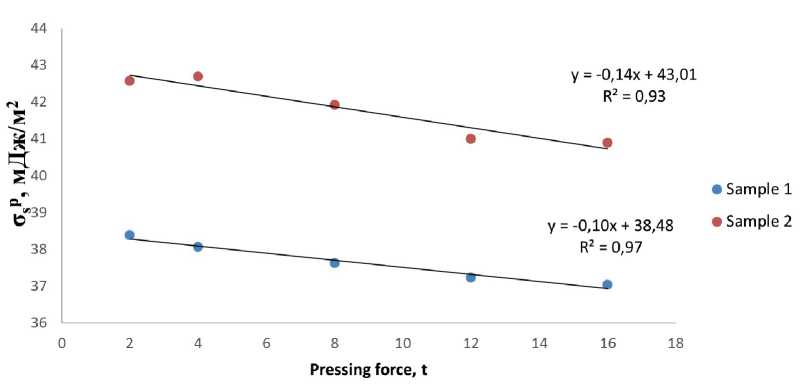
Fig. 6. Functional dependence of the form σsp = f (T) for concrete powders
Table 4
Physicochemical and energy characteristics of powders
Powder |
σ0 • 103, J/m2 |
Sуд, m2/kg |
ES • 10–4, J/kg |
Еm • 10–6, J/kg |
kS • 103 |
Concrete (sample 1) |
60.23 |
5000 |
0.030 |
4.3 |
0.070 |
Concrete (sample 2) |
65.45 |
5150 |
0.034 |
3.6 |
0.094 |
CONCLUSIONS
The possibility of calculating the macroenergy characteristics (atomization energy, specific mass atomization energy) of waste concrete samples using the results of X-ray fluorescence analysis is shown. It was found that, despite the similarity of the elemental composition, concrete with a residual strength class B15 has a higher value of the atomization energy. This physicochemical indicator characterizes the potential reserve of internal energy of the system, which is capable of transforming into free surface energy during mechanical destruction of the material.
To assess the activity of dusty fractions of concrete waste as a component in binding compositions of the hydration type of hardening, it is proposed to use the value of the surface activity of powder systems as a criterion. It has been established that for concrete waste the value of this criterion is determined by the concrete strength class.
MANUFACTURING TECHNOLOGY FOR BUILDING MATERIALS AND PRODUCTS
Список литературы Surface activity of concrete waste powders
- Efimenko A.Z. Waste concrete is a raw material for the production of efficient building materials. Concrete Technologies. 2014;(2):17–21.
- Gusev B.V., Kudryavtseva V.D., Potapova V.A. Concretes with nano-additive from fired reclaimed concrete. Nanotechnologies in Construction. 2020;12(5):245–249. Available from: doi: 10.15828/2075-8545-2020-12-5-245-249.
- Krasinikova N.M., Khozin V.G. Recycling of concrete scrap as raw materials for cement concrete. Construction Materials. 2020;1(2):56–65. Available from: doi: 10.31659/0585-430X-2020-778-1-2-56-65.
- Lesovik R.V., Akhmed A.A.A., Alaskhanov A.Kh. Binder from the dusty fraction of fragments of destroyed buildings and structures in Iraq. Regional Architecture and Engineering. 2020;1(42):69–76.
- Gusev B.V., Krivoborodov Yu.R., Potapova V.A. Possibility of secondary use of concrete breaker. Technique and technology of silicates. 2020;27(1):28–31.
- Lesovik R.V., Ahmed A.A.A., Al Mamouri S.K.S., Gunchenko T.S. Composite binders based on concretes crap. Bulletin of BSTU named after V.G.Shukhov. 2020;7:8–18. Available from: doi: 10.34031/2071-7318-2020-5-7-8-18.
- Abramovskaya I.R., Aizenshtadt A.M., Frolova M.A., Veshnyakova L.A., Tutygin A.S. Energy of highly dispersed composites of rocks. Nanotechnologies in Construction. 2013;(3):56–65.
- Veshnyakova L.A., Aizenshtadt A.M., Frolova M.A. Evaluation of the surface activity of highly dispersed raw materials for composite building materials. Physics and chemistry of materials treatment. 2015;(2):68-72.
- Veshnyakova L.A., Drozdyuk T.A., Aizenshtadt A.M., Frolova M.A., Tutygin A.S. Surface activity of siliconcontaining rocks. Material Science. 2016;(5):45-48.
- Morozova M.V., Akulova M.V., Frolova M.A., Schepochkina Yu.A. Determination of energy parameters of sands on the example of deposits in the Arkhangelsk region. Material Science. 2020;(9):45–48. Available from: doi: 10.31044/1684-579X-2020-0-9-45-48.
- Yakovets N.V., Krutko N.P., Opanasenko O.N. Determination of free surface energy of powdered resinousasphaltene substances by the Owens-Wendt-Rabel-Kaelble method. Sviridovskiye chteniya. 2012;(8):253 – 260. (In Russian).
- Danilov V.E., Ayzenshtadt A.M., Frolova M.A., Tutygin А.S. Dispersion Interactions as Criterion of Optimization of Cementless Composite Binders. Inorganic Materials: Applied Research. 2018;9(4):767–771.
- Danilov V.E., Korolev E.V., Aizenshtadt A.M. Measurement of contact angles of powder wetting by the “sessile drop” method. Physics and chemistry of materials treatment. 2020;(6):75–82. Available from: doi: 10.30791/0015-3214-2020-6-75-82.
- Danilov V.E., Ayzenstadt A.M. Comprehensive approach to the assessment of nanosized fractions of polydisperse systems of crushed rocks. Nanotechnologies in Construction. 2016;8(3):97–110. Available from: doi: 10.15828/2075-8545-2016-8-3-97-110.
- Gregg S.J., Sing K.S.W. Adsorption, Surface Area and Porosity. 2nd ed. London: Academic Press; 1982.
- Almyasheva O.V., Fedorov B.A., Smirnov A.V., Gusarov V.V. Size, morphology and structure of particles of zirconium dioxide nanopowder obtained under hydrothermal conditions. Nanosystems: Physics, Chemistry, Mathematics. 2010;1(1):26–36.
- Zuev V.V., Potselueva L.N., Goncharov Yu.D. Crystal energy as a basis for assessing the properties of solid materials. Saint-Petersburg: Publishing House of the Factory “Al’fapol”; 2006.
- Abramovskaya I.R., Aizenshtadt A.M., Lesovik V.S., Veshnyakova L.A., Frolova M.A., Kazlitin S.A. Calculation of the energy intensity of rocks – as raw materials for the production of building materials. Industrial and Civil Engineering. 2012;(10):23–25.
- Binnewies M., Milke E. Thermochemical Data of Elements and Compounds. Hannover: Wiley-VCH; 2002.
- Gurvich L.V., Veits I.V., Medvedev V.A. and etc. Thermodynamic properties of individual substances: a reference book. Volume IV. Moscow: Nauka; 1982.
- Gurvich L.V., Veits I.V., Medvedev V.A. and etc. Thermodynamic properties of individual substances: a reference book. Volume III. Moscow:Nauka;1982.
- Gurvich L.V., Veits I.V., Medvedev V.A. and etc. Thermodynamic properties of individual substances: a reference book. Volume II. Moscow: Nauka; 1982.
- Gusev B.V., Kudryavtseva V.D. Crushing of reinforced concrete products and recycling of concrete. Technique and technology of silicates. 2013;20 (2):25–28.
- Galkin Yu.Yu., Udodov S.A. Phase analysis of the structure of cement stone isolated at its early loading. Russian journal of transport engineering. 2018;1(5). Available from: https://t-s.today/PDF/21SATS118.pdf [Accessed 10th April 2021]. Available from: doi: 10.15862/21SATS118.
- Khabas T.A., Kulinich E.A., Egorov E.Yu. Thermogravimetric method for the analysis of silicate materials. Guidelines for the courses “Physicochemistry of a solid” and “Physical chemistry of refractory non-metallic and silicate materials.” Tomsk: TPU; 2007.
- Agzamov F.A., Lomakina L.N., Gafurova E.A., Bikmeeva N.B. Investigation of the process of structure formation of concrete in the conditions of winter concreting. Oil and Gas Business. 2013;(6):384–400.
- Gayda J., Ayzenshtadt A., Tutygin A., Frolova M. Organic-Mineral Aggregate for Sandy Subsoil Strengthening. Procedia Engineering. 2016;143:90–97.
- Levin A.V. Basic principles of particle size analysis. GK “Energolab”. Available from: https://docplayer.ru/43708708-Osnovnye-principy-analiza-razmerov-chastic.html [Accessed 10th April 2021].